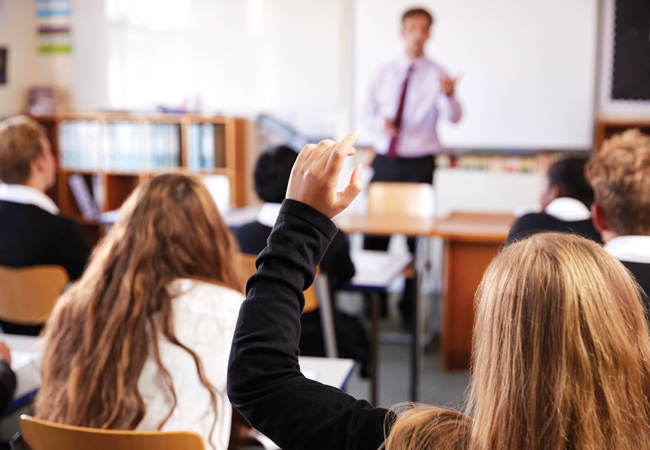
Credit: iStock
School buildings in the UK account for 2% of national carbon emissions, and half the emissions of local authorities. To meet national climate-change mitigation targets, the energy efficiency of new and existing school buildings needs to increase. This could be achieved through the adoption of voluntary energy efficiency standards, such as Passivhaus, which has a fabric-first approach and clearly defined certification criteria.
In the meantime, there is mounting evidence on the adverse effects of poor indoor air quality (IAQ) on children’s health and on their cognitive performance and ability to concentrate. Published evidence on the IAQ of UK schools is limited, however, and scarce for Passivhaus schools, in particular. To address this evidence gap, the IAQ of two UK Passivhaus primary schools was monitored.
School A was completed in 2013 and is in a suburban area. Balanced supply and extract rates are dictated by return air CO2 levels. Fresh air is supplied to the classrooms, then ‘used’ air is displaced to the ‘hub space’ (circulation and activities space) through sound-attenuated air paths.
Extraction is through the hub space and WCs. School B was completed in 2015 and is in an urban location. Supply and extract rates in the classrooms are fixed, and inlets/outlets are located in the classrooms. Heat recovery is used in winter, while a mixed-mode ventilation strategy is employed in summer, with users free to open windows.
In both schools, natural ventilation is achieved with user-operated windows and grilled ventilation panels in classrooms. Both have high-level windows in the hub (School A) or corridor (School B) of the first floor. These are operated by a BMS with override switches in School A, and user-operated in School B. School A has no high-level windows in classrooms.
All first-floor classrooms in School B have user-operated high-level windows. Airtightness is less than 0.6 air changes per hour at 50Pa. The design target ventilation rates were in line with ‘current at the time of completion’ guidance.
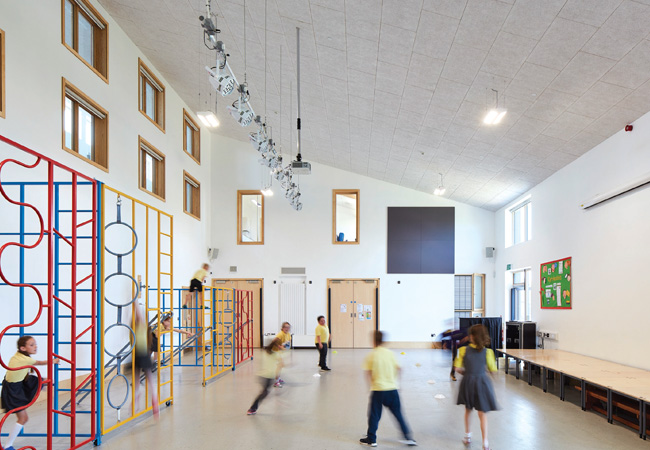
Trimsaran Primary School is a Passivhaus school, but not one of those studied in this research
In each school, monitors were installed in three classrooms and at an outdoor location on the roofs of the main buildings. Real-time monitoring took place at five-minute intervals between September 2018 and July 2019 for dry bulb temperature (DBT), relative humidity (RH), carbon dioxide (CO2) and particulate matter (PM2.5). In addition, passive diffusive sampling of nitrogen dioxide (NO2), and total and targeted volatile organic compounds (VOC) took place over a period of four days – including unoccupied hours – during winter and summer.
While these initial results should be interpreted with caution, given the study’s small sample size, the indoor environmental variable ranges were within the range reported in previous studies of UK schools, such as the UK part of the Schools Indoor Pollution and Health: Observatory Network in Europe project (SINPHONIE-UK).
All classrooms met the overheating risk criteria based on adaptive thermal comfort thresholds during the summer monitoring period. The winter upper static threshold was slightly exceeded in four classrooms, all of which were on the first floor and had south-facing windows (low or high level) combined with minimal shading. The combination of these features can account for higher classroom temperatures in both seasons.
The differences in classroom CO2 concentration between the two schools in winter reflected the different ventilation strategies and design targets in line with requirements at the time the schools were built (Figure 1). All averages were significantly lower than those in existing studies in UK primary school buildings (for example, SINPHONIE-UK). While further increasing ventilation rates is likely to dilute CO2 to concentrations below the current regulatory requirements in School A, this could also lower relative humidity to unacceptable levels and increase energy use. Lower set points for acceptable CO2 levels, and lower air supply temperatures, could be investigated.
Similar to CO2, total VOC (TVOC) levels were higher in winter than in summer (Figure 2). Ventilation systems in School A were found to be less effective in diluting TVOC in winter compared with School B. With regard to specific VOC, monitoring results indicate that limonene and pinene levels are consistent with average levels in public buildings and schools in the UK, and that they were introduced in the classroom through strong indoor sources. These two terpenes are associated with intermittent indoor pollution, such as cleaning, and effectiveness of dilution during and after cleaning. It should be investigated whether ventilation rates during and immediately after cleaning (morning for School B and evening in School A) could influence the contribution of cleaning procedures to indoor air pollution.
For VOC emitted from cleaning, children are probably exposed to lower concentrations than those reported in this study, because monitoring included after-class hours, when children were not in the school and cleaning took place.
The VOC 2-ethyl-hexanol was detected in winter in all three classrooms in School A (average 3.6μg.m-3). This is below the level of interest and was not detected in outdoor samples, summer samples, or any School B samples. Its presence can be linked to lingering effects from wall painting in School A that took place no more than nine months before winter sampling.
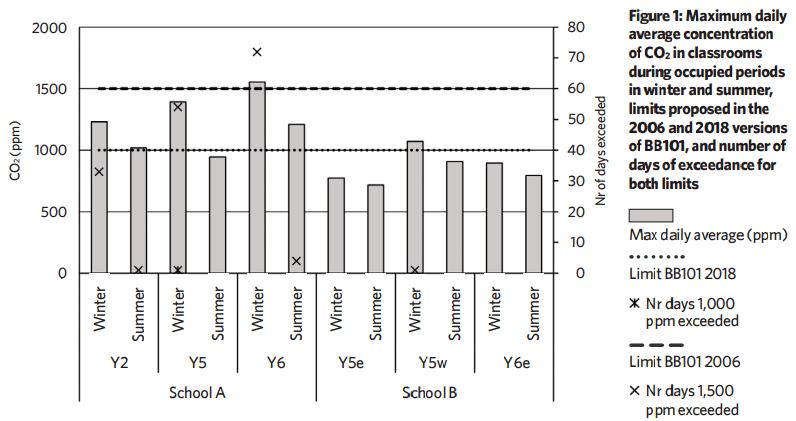
Indoor benzene levels in schools A and B (averages 0.80μg.m-3 and 0.64μg.m-3 in winter and below 0.32μg.m-3 in summer) were consistent with indoor benzene levels reported for UK primary schools. Analysis of the indoor/outdoor ratios in the existing study suggested indoor sources, such as arts and crafts materials, but also adhesives from building materials and carpets. Benzene and NO2 (not filtered in schools) depend on the infiltration of the pollutants from outdoors. The location of the school in relation to busy roads and the prevailing wind direction must be considered at design stages. Differences in NO2 indoor/outdoor ratios in the two schools can result from different ventilation rates and the reaction rate of the compound with indoor surface materials .
Classroom average PM2.5 levels across both schools were 4.1μg.m-3 in winter and 3.3μg.m-3 in summer (Figure 3). The PM2.5 indoor/outdoor ratios reported in this study (0.3-0.4 in winter and 0.4-1.0 in summer) were lower for winter than those in previous studies with predominantly naturally ventilated school buildings in Europe (for example, SINPHONIE-UK). The findings are in agreement with studies suggesting that mechanically ventilated buildings are likely to have lower PM2.5 infiltration rates than naturally ventilated buildings.
The adaptive temperature criteria for the assessment of summertime overheating risk were met in all classrooms in the investigated schools, but higher temperatures in both seasons are associated with south-facing windows with limited shading. Solar control for summer should be designed for south-facing windows, to ensure better control of summertime overheating, at higher outdoor temperatures, when ventilation cooling is less effective.
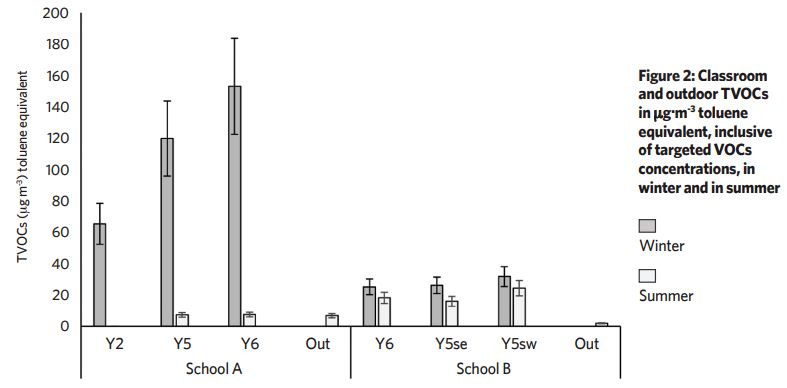
Differences between the two schools, designed to meet different daily average supply rates corresponding to the different CO2 compliance criteria in two versions of the Building Bulletin BB101 Ventilation, thermal comfort and indoor air quality (2006 and 2018), show that higher ventilation rates can limit indoor-sourced pollutants, such as CO2 and VOC. For building facilities managers (FMs), this means liaising with the cleaning and teaching teams, school management and controls engineers to adjust the ventilation schedule to account for dilution of pollution events.
In very airtight buildings, such as Passivhaus schools, the infiltration of outdoor-sourced pollutants, such as NO2 and benzene, can possibly be controlled through ventilation strategies that take into account the position of the air inlet in relation to pollution sources and prevailing wind direction.
Mechanical ventilation and mixed-mode ventilation strategies with filtration combined with an airtight building envelope, can provide more effective protection from exposure to PM2.5 in polluted environments.
Mechanical ventilation strategies in Passivhaus schools are required to balance not only indoor sources, but also the fluctuations of outdoor air pollution. Strategies for good IAQ during operation should employ increased monitoring of indoor and outdoor air-pollution levels for adaptive adjustment of the ventilation schedule. CJ
■ Chryssa Thoua, Dr Anna Mavrogianni and Proffesor Dejan Mumovic, Institute for Environmental Design and Engineering, UCL, Lia Chatzidiakou, Department of Chemistry, University of Cambridge, Mark Lumley, associate director, Architype