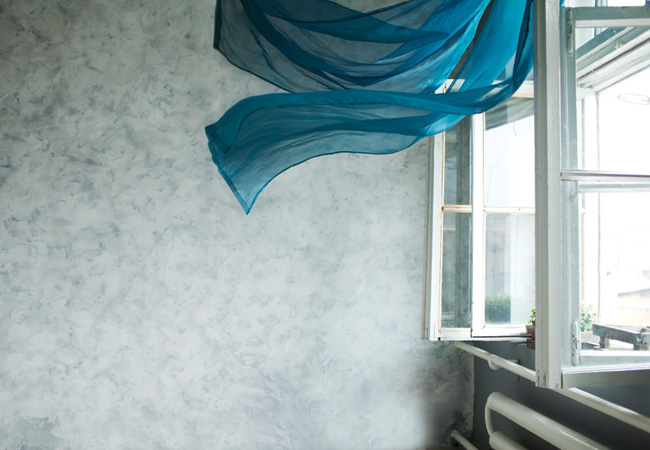
Credit: iStock/Roddy1red
In a recent review of 31 green-building certification schemes used around the world, indoor air quality (IAQ) was found to contribute to just 7.5% of the final score on average.1 As policy-makers strive to reduce the energy demands of buildings by sealing or reducing outdoor air ventilation rates, an unintended consequence could be a reduction in the quality of indoor air, with corresponding negative health effects for occupants. This article summarises the discussions had during an Air Infiltration and Ventilation Centre (AIVC) workshop on IAQ metrics, held in Brussels, Belgium, in March.2 It identifies the types of contaminants found in buildings today, the mechanisms of exposure to them, and the methods of mitigating their effects. It also explores metrics that could be used to quantify the quality of indoor air.
Problems
Building materials and systems – and activities in buildings – can be sources of contaminants harmful to human health. Some materials used to construct and furnish buildings emit harmful gases and harbour biological organisms. Unvented combustion processes for space and food heating emit gaseous and particulate contaminants, and can be a source of moisture that is a primary driver of biological growth. Human activities – such as cooking and vacuuming – also discharge particulates; cleaning and deodorising products give off gaseous contaminants and particulates; and smoking emits more than 7,000 compounds, many of which are harmful.3 Pets conceal biological contaminants, and can themselves be allergens. People and pets also emit gaseous bio-effluents that smell, and harbour pathogens that produce disease.
The presence and concentrations of airborne contaminants are often measured without careful consideration of their relevance. Some are inappropriately grouped together; for example, more than one million volatile organic compounds (VOCs) with unknown toxicities exist – yet they are sometimes reported as single values, and referred to as total VOCs (TVOCs).
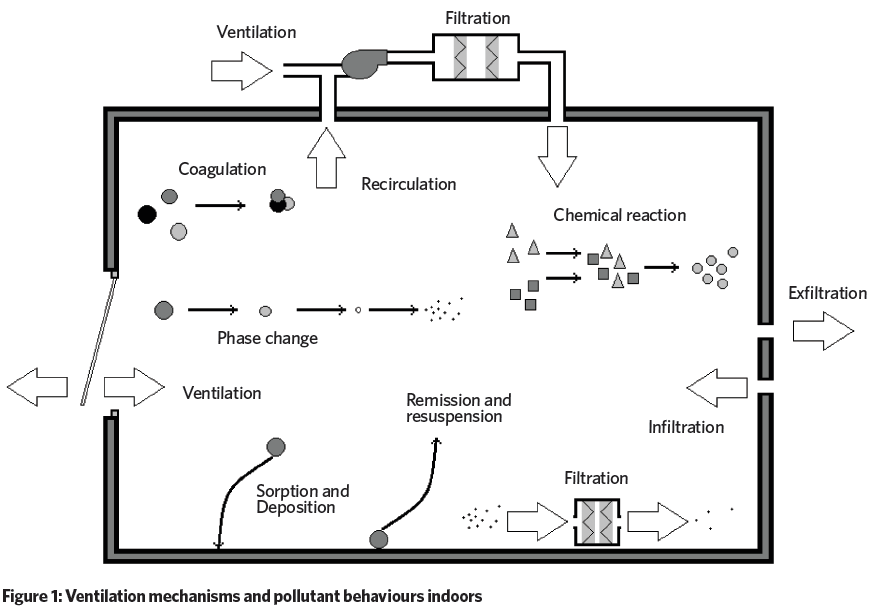
Carbon dioxide (CO2) is often used as an indicator of poor IAQ; although it does not negatively affect the health of occupants in the concentrations usually found in buildings, it is a marker of human bio-effluents. Its presence is a function of: occupancy; occupant activity, gender, age and physiology; combustion; and transport from elsewhere. Without an understanding of these variables, indoor CO2 cannot be used to assess IAQ or ventilation. And it can never be used to indicate the presence of other important indoor contaminants – such as formaldehyde from building materials, the emission of which is unrelated to CO2 concentration.
However, existing measurements of contaminants – the type and toxicity of which are known – still give cause for concern.4 They could negatively affect the health of occupants and – when extrapolated to larger building stocks – could adversely affect healthcare systems and economies.
What do we know about IAQ?
Ventilation standards generally agree that indoor air should be perceived as fresh and pleasant by the majority of occupants, so they set a baseline ventilation requirement of around 8 L.s-1 per person to dilute bio-effluent odours to an acceptable level for anyone entering an occupied room from relatively clean air.5 They then attempt to account for other contaminants by increasing the baseline rate to around 10 L.s-1 per person, although the increase is not based on specific contaminants.6
Ventilation rates in national standards around the world differ by up to four times, and their origins aren’t always known or documented.7 Comparisons of measured ventilation rates against those prescribed by national standards suggest there is also a widespread inability to implement them effectively in many buildings,8 such as houses9 and schools.10
The ability of ventilation to mitigate these contaminant exposures is limited. Occupants are exposed to contaminants via: inhalation, dermal absorption (through the skin), and ingestion. Infections – carried by fomites such as skin cells, hair, clothes, bedding and furniture – and are spread by all three. The pumping action of doors, the movement of bedding, and sitting on soft furniture can all resuspend fine particles (see Figure 1), which can be inhaled into the lower respiratory tract. Large droplets produced by breathing, talking, sneezing, and coughing contain mucus, saliva, cells, and infectious agents that are transmitted over distances of less than one metre. Such particles can be inhaled into the upper respiratory tract.11
Semi-volatile organic compounds (SVOCs), such as those emitted by dry-cleaned clothing or flame retardants, are absorbed through the skin or by food and ingested.12 Organic allergens – produced by dust mites, for example – are in bedding, carpets, and soft furnishings, and are inhaled.13 On its own, ventilation is insufficient, and fails to deliver acceptable IAQ, especially when contaminant sources are not reduced or eliminated.
Practical solutions
By the mid-1800s, pioneer of modern hygiene and environmental science Max Joseph von Pettenkofer identified source control as the most effective step towards acceptable IAQ.14
When source control is impossible, local exhaust ventilation – such as a kitchen cooker hood – is effective in removing contaminants before they mix in a space. These devices are imperfect, so diluting well-mixed contaminants using ventilation, or removing them with an air cleaner, is important. Although a useful alternative to ventilation, these devices have energy and financial penalties, as well as performance limitations. There is also evidence that they can re-emit collected particulates, and serve as sites for microbiological growth or chemical reactions that create secondary contaminants, such as ozone, formaldehyde, and other VOCs.15 There is a pressing need for standardisation and performance data for these devices.
Some contaminants, such as carbon monoxide (CO), are harmful when exposure is acute, so sensors and alarms can be useful for monitoring levels. However, many others require exposures to be chronic before negative health effects occur. Devices indicating the presence of specific contaminants should be used.
IAQ metrics
An air-quality metric should identify when the quality of indoor air is unacceptable, and should be based on its effects on human health and comfort, acknowledging that they may not be immediate.
One method is to ask occupants. The human nose is as sensitive to some gaseous contaminants as chemical analyses; using it indicates occupant preference and ensures people are the focus of an assessment. Perceived air quality (PAQ) is the basis of most ventilation standards and is used to assess indoor odours16 and IAQ.17 However, its subjectivity, humans’ inability to smell all harmful contaminants, its high dependence on temperature and relative humidity,18 and the propensity of people to adapt to malodours after only a few minutes,19 are concerning.
Another method is to identify properties of a building that are known to affect IAQ directly – for example, by applying a tick-box approach. Each feature could be weighted according to its hazard and aggregated to produce a single metric. This method could help develop a third-party rating system – similar to many energy-rating schemes – and help people sensitive to specific contaminants when choosing a house.
“Ventilation rates in national standards around the world differ by up to four times”
To obtain a comprehensive picture of the IAQ in a building, we need to measure a range of contaminants. However, their concentrations may be incomparable because of varying health impacts and time scales, and different units – for example, radon (Bq.m-3) and particulate matter (μg.m-3). One approach is to convert the individual contaminant concentrations into sub-indices – which may be a function of their health risks – before they are aggregated into a single index. Although the summing of sub-indices can lead to situations where they are all under individual health thresholds, the final index shows exceedance. Conversely, the averaging of sub-indices can lead to a final index that indicates acceptable IAQ when one or more sub-indices is greater than their individual thresholds. One solution is to use the maximum of all sub-indices as the final index,20 but this does not indicate overall IAQ. Other methods weight the sub-indices before aggregation.21
Exposure limit values (ELV) are used in occupational environments to prevent or reduce risks to health from hazards – such as vibrations22 – by setting a maximum quantity experienced per person per day. This principle could be applied when measuring the concentrations of a range of contaminants in a building. Here, the ratios of their maximum concentrations to their respective ELV concentrations give a quick indication of risk, where a ratio <<1 might be acceptable, but one approaching or exceeding unity may be problematic.
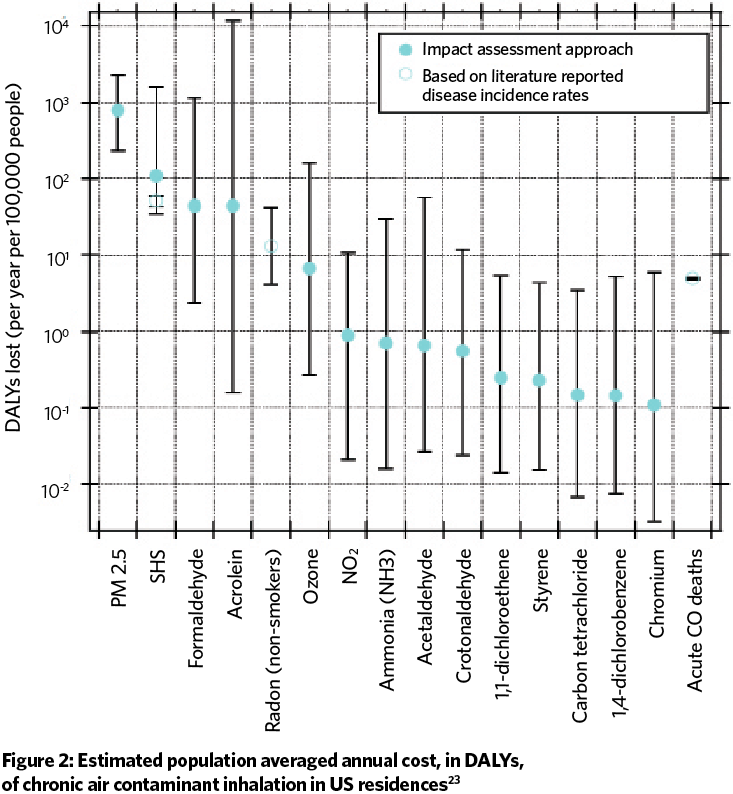
A problem with IAQ indices and ELVs is that it isn’t clear how a change to either metric – say by 10% – would affect occupant health and comfort. An indication of the relationship between exposure and health consequences is required.
The disability adjusted life year (DALY) is a measure of time, where a value of unity is one year of healthy life lost to disease or injury. DALYs are calculated as the sum of years of life lost to premature mortality and morbidity in a population for some negative health effect. Disability is weighted by its effect on people’s life in general, accounting for mental illness. In the case of IAQ, the burden of disease is a measure of the difference between the current health status of building occupants and an ideal situation where they all live into old age, free of disease and disability. The DALY has been used by the AIVC23 to prioritise indoor contaminants found in houses for mitigation (see Figure 2).
Next steps
Metrics must be trustworthy because unreliable evidence can be disputed and could lead to litigation. A metric must have robust technical specifications, prescribing the methods of measurement and calculation. It must clearly identify measurement locations, device types, tolerances, calibration intervals, and measurer and analyst competences. This will increase the likelihood that two assessors surveying the same building arrive at the same score.
Metrics should not be a barrier to innovation, so it is important that methods of pollution control are not prescribed. Any remediation measure should consider the need simultaneously to provide acceptable IAQ and energy-use reduction (see Figure 3), so they should only be used when they are effective in achieving both. This requires good sensing and control devices. When non-compliance is identified, pre-defined sanctions must be imposed.
The consideration of IAQ and its effects on occupant health and comfort will lead to a new paradigm in building standards and guidelines, moving them beyond the control of odour towards the provision of indoor environments that consider occupant health. ASHRAE 62.224 has begun this transition, and – as other standards join – they will start to have a tangible effect on people, healthcare systems and economies. CJ
- The 38th AIVC conference will be held on 13-14 September in Nottingham.
- Dr Benjamin Jones is assistant professor at the Faculty of Engineering, University of Nottingham. The author is grateful to Max Sherman, Andrew Persily, Iain Walker and Rémi Carrié for their comments on this article.
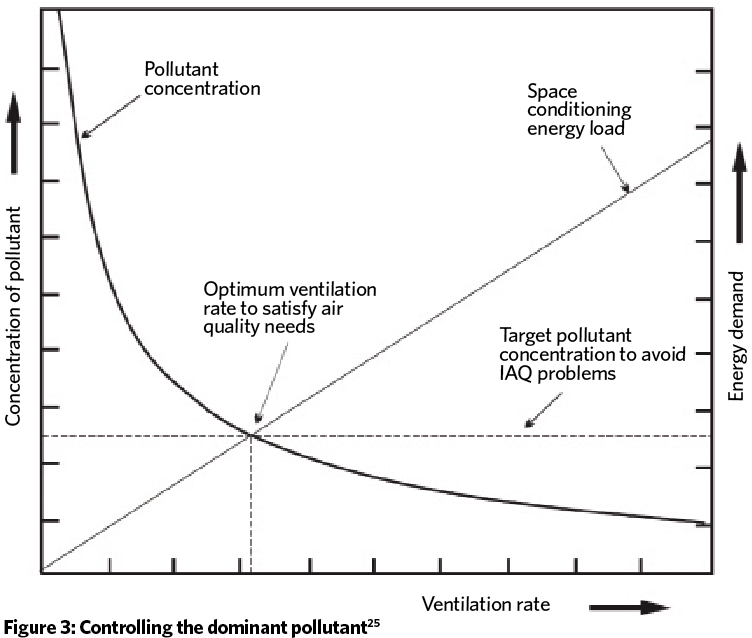
References
- Wei W, Ramalho O, Mandin C. Indoor air quality requirements in green building certifications. Building and Environment. 2015;92:10-9.
- AIVC. Is ventilation the answer to indoor air quality control in buildings? Do we need performance- based approaches? AIVC Workshop held in Brussels, Belgium. 14th-15th March, 2017.
- CfDC. How Tobacco Smoke Causes Disease. The Biology and Behavioral Basis for Smoking- Attributable Disease. Centers for Disease Control. Atlanta, Georgia, U.S.A.: U.S. Public Health Service; 2010.
- Logue JM, McKone TE, Sherman MH, Singer BC. Hazard assessment of chemical air contaminants measured in residences. Indoor Air. 2011;21:92-109.
- Persily A. Challenges in developing ventilation and indoor air quality standards: The story of ASHRAE Standard 62. Building and Environment. 2015;91:61-69.
- Persily A. What We Think We Know about Ventilation. International Journal of Ventilation. 2006;5:275-90.
- Borsboom, W. Rational Behind Current Ventilation Airflow Rates. Presentation to AIVC Workshop: Is ventilation the answer to indoor air quality control in buildings? Do we need performance-based approaches? Brussels, Belgium. 14th March, 2017.
- Persily A. Field measurement of ventilation rates. Indoor Air. 2016;26:97-111.
- Dimitroulopoulou C. Ventilation in European dwellings: A review. Building and Environment. 2012;47:109-25.
- Chatzidiakou L, Mumovic D, Summerfield AJ. What do we know about indoor air quality in school classrooms? A critical review of the literature. Intelligent Buildings International. 2012;4:228-59.
- Atkinson J, Chartier Y, Pessoa-Silva CL, Jensen P, Li Y, Seto W-H. Natural Ventilation for Infection Control in Health-Care Settings. World Health Organization; 2009.
- Weschler CJ, Nazaroff WW. Semivolatile organic compounds in indoor environments. Atmospheric Environment. 2008;42:9018-40.
- Biddulph, P et al. Predicting the population dynamics of the house dust mite Dermatophagoides pteronyssinus (Acari: Pyroglyphidae) in response to a constant hygrothermal environment using a model of the mite life cycle. Experimental and Applied Acarology. 2007;41:61-86.
- Fanger PO. What is IAQ? Indoor Air. 2006;16:328-34.
- Siegel JA. Primary and secondary consequences of indoor air cleaners. Indoor Air. 2016;26:88-96.
- ISO. 16000-30 Indoor air: Sensory testing of indoor air. International Standards Organization. 2014.
- Wargocki P, Fanger PO, Krupicz P, Szczecinski A. Sensory pollution loads in six office buildings and a department store. Energy and Buildings. 2004;36:995-1001.
- Berg-Munch B, Clausen G, Fanger PO. Ventilation requirements for the control of body odor in spaces occupied by women. Environment International. 1986;12:195-9.
- Fang L, Clausen G, Fanger PO. Impact of Temperature and Humidity on the Perception of Indoor Air Quality. Indoor Air. 1998;8:80-90.
- Sharma M, Bhattacharya A. National Air Quality Index. Central Pollution Control Board. Ministry of Environment, Forests, & Climate Change; 2012.
- Abadie M, Wargocki P, Rode C. IEA EBC Annex 68 – Indoor Air Quality Design and Control in Low Energy Residential Buildings – Setting the Metrics. The 14th international conference of Indoor Air Quality and Climate. Ghent, Belgium. July 3-8, 2016.
- HSE. Hand-arm vibration. – The Control of Vibration at Work Regulations 2005. Health and Safety Executive. ISBN 0-7176-6125-3. Richmond, UK. 2008.
- AIVC. TN 68: Residential Ventilation and Health. Air Infiltration and Ventilation Centre, Brussels, Belgium; 2016.
- ASHRAE. ANSI/ASHRAE Standard 62.2 – Ventilation and Acceptable Indoor Air Quality in Residential Buildings. 2016.