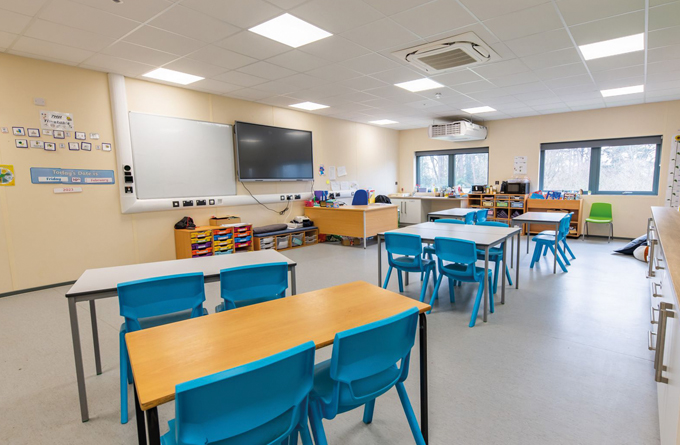
The overall performance of mechanical ventilation and heat recovery (MVHR) units is dependent on a combination of (sometimes opposing) requirements that need to be holistically considered to determine the correct application. This CPD will explore the sensible heat recovery efficiencies required from MVHR units for a variety of modelled commercial and institutional building applications in a temperate climate, in order to better understand what should be applied for optimum wintertime performance.
Driven by legislative obligations, such as the Ecodesign regulations, and the escalating necessity of environmental responsibility, building owners and industry professionals are increasingly installing some form of heat recovery device on all sizes of ventilation systems.
The ecodesign requirements for MVHR
Great Britain (England, Scotland and Wales) embodied the EU requirements in the UK Ecodesign for Energy-Related Products and Energy Information Regulations 2021 (ErP 2021), so practically the EU regulations have relevance across the whole of geographical Europe.
EU 1253/20141 defines the required performance for non-residential MVHR units that in typical conditions require a minimum thermal efficiency of 73% for plate heat exchangers and thermal wheels, and 67% for a run-around HRS. The basic maximum internal specific fan power (SFP) for the unit is also specified, but with a higher efficiency HRS this may be increased, so encouraging the use of a more efficient HRS.
In the typically compact form that makes up a MVHR system (as in the example shown in Figure 1), the type of heat recovery that is generally employed is a plate heat exchanger. Some systems employ heat pipes for heat recovery, and in larger MVHR units it would not be unusual to see a thermal wheel or, occasionally, a run-around coil.
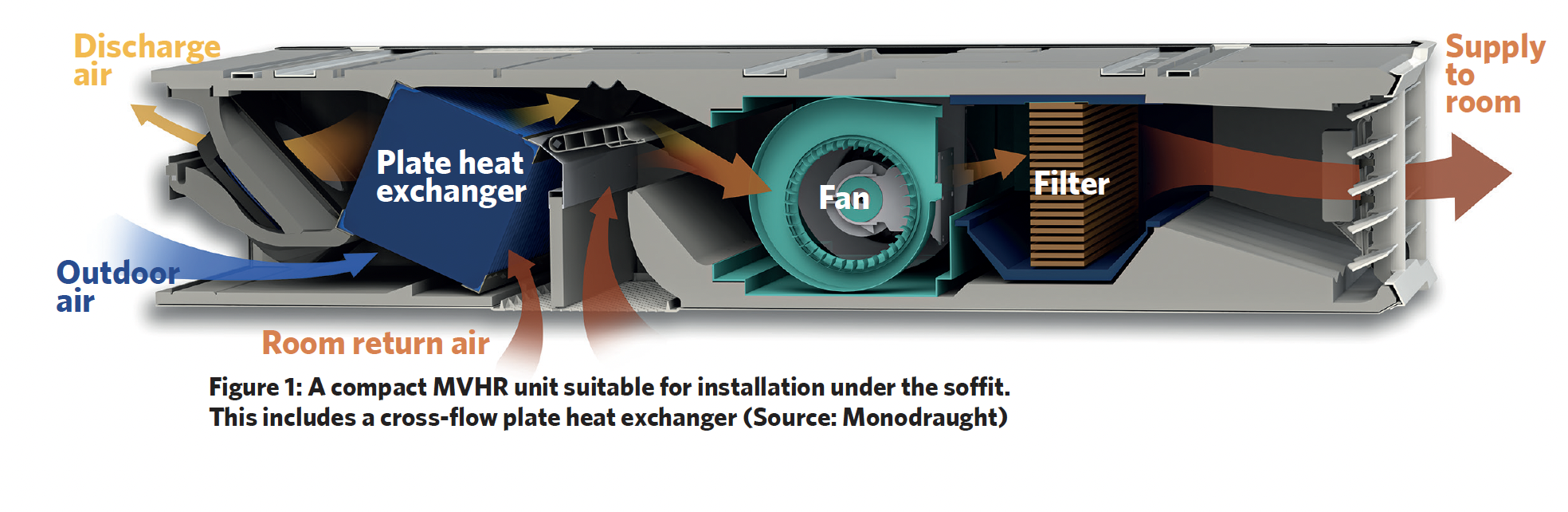
During winter, an occupied building’s indoor air is heated by the casual gains of occupants, appliances, lighting, equipment and solar gain, as well as any heating system. An MVHR unit is primarily used to deliver ventilation air from outdoors to maintain good indoor air quality (IAQ).
The heat exchanger in the MVHR transfers heat from a building’s vitiated exhaust air to the incoming outdoor air, to raise (or lower) the temperature – and, in some cases, moisture content – of the incoming air. This reduces or negates the need to add, or remove, sensible and, possibly, latent heat.
When designed, installed and operated appropriately, these can deliver significant life-cycle energy savings, since the power required to operate the heat exchangers – normally as a result of the need for additional fan power, as the heat exchanger adds resistance to the airflow path – can be substantially lower than the power that is gained from the transfer of heat between the fresh air and the exhaust air.
Systems with high heat recovery efficiency tend to have a much lower ventilation flowrate efficiency, as the heat exchangers create significant resistance to airflow. This means that when the heat recovery is not fully required or used, the efficiency of the systems suffers owing to the increased resistance to airflow. Many ventilation systems have a bypass airpath to avoid the heat exchanger; however, these are typically sized with the same flowrate efficiency as through the heat exchanger.
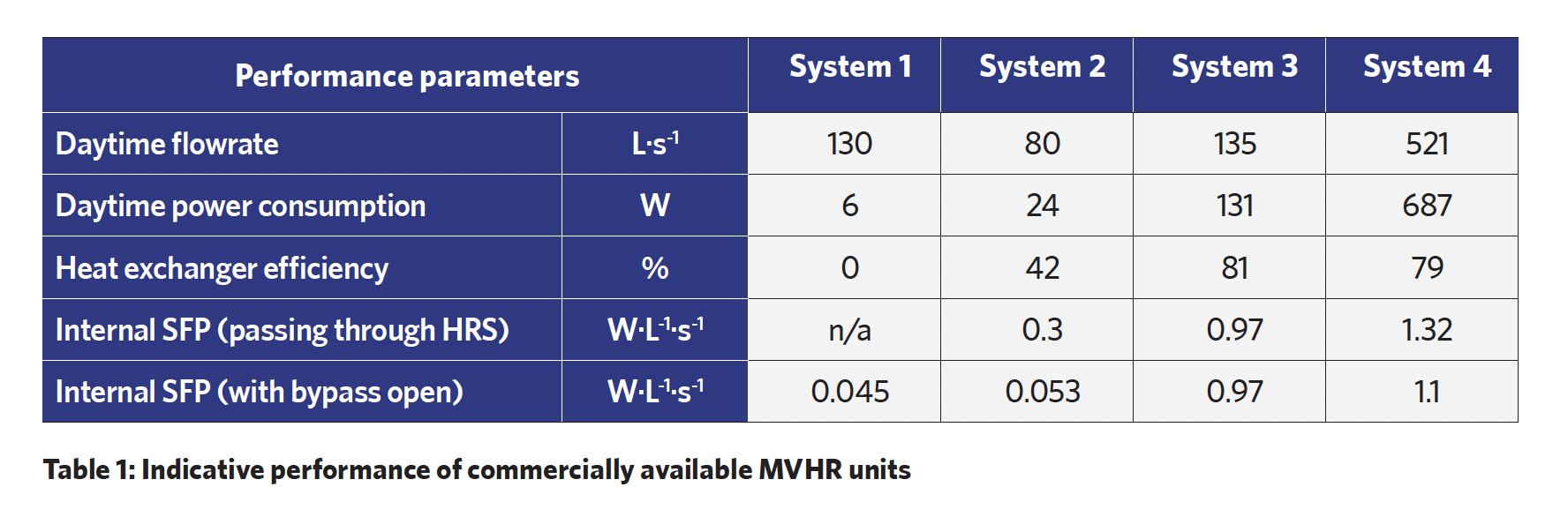
Table 1 shows data taken for some example commercial MVHR systems, representing efficiencies for systems of their type. System 1 is a simple balanced flow mechanical ventilator with no HRS; system 2 has a low-efficiency heat exchanger; systems 3 and 4 have high-efficiency heat exchangers (but also include a heat exchanger bypass that has an airflow resistance that almost matches that of the heat exchanger).
The impact of the HRS on the SFP is clear. At one extreme, the system with no HRS can provide ventilation while consuming under 5% of the (internal) fan power compared with the units with a high-efficiency HRS. Systems with lower heat exchanger efficiency have the lower internal SFP. This property of MVHRs seeded the idea for the study that is reported in this CPD, which was undertaken by Kieran Kilgariff, of Monodraught. His study sought to explore the optimum HRS efficiency for a number of example MVHR applications while maintaining room IAQ through supplying outdoor air.
To provide some nominal, but reasonably realistic, assessment of ventilation needs, Kilgariff created some simple room models. These included several building room types drawn from the secondary education sector – a general classroom, a science lab, an assembly hall, and a sports hall. Each room was modelled with three different occupancy levels (low, medium and high) to represent typical use cases. For example, the classroom at low occupancy represents a classroom at a special educational needs and disabilities (SEND) school; the sports hall at low occupancy represents a typical physical education lesson and, at high occupancy level, represents it being used as an exam room.
There were three commercial building room types – a large open-plan office space, a small personal office or meeting room, and a boardroom or large meeting room. Each room variant was modelled with three different occupancy levels representing the occupancy densities given by CIBSE Guide A2 for these room types.
Each educational and commercial room type was modelled with three different building constructions to represent buildings with different thermal performances. Room type 1 represented a room with minimal external façades and glazing; type 3 a room with a high level of external façades and glazing; while type 2 represented a middle ground between the two. Fabric thermal performance was taken from limiting values of the 2021 edition of England Building Regulations AD L Volume 2.3
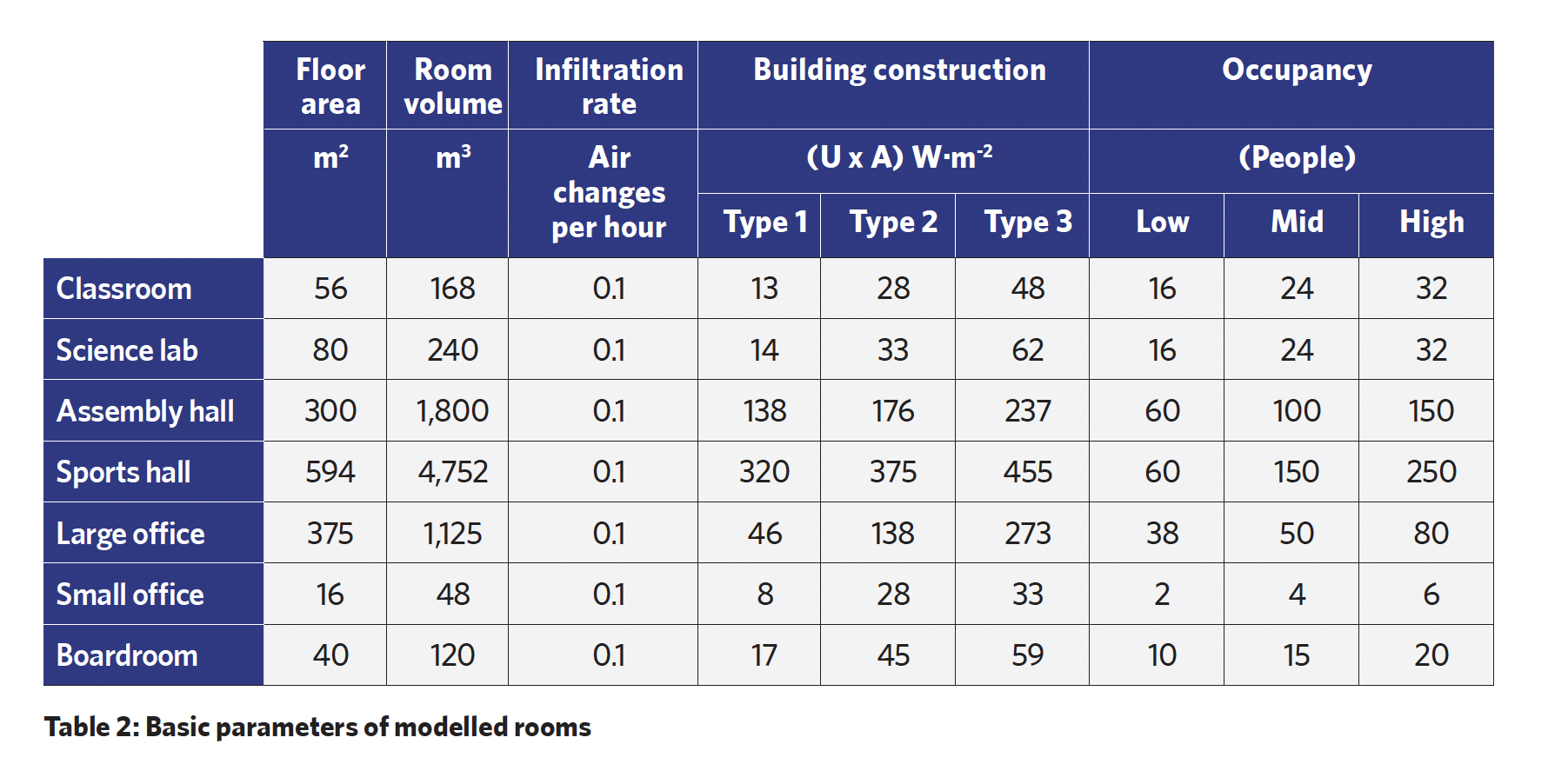
Table 2 provides a summary of the rooms modelled alongside their building constructions (represented by the sum of the U-values multiplied by external area) and occupancy levels.
Each room was modelled using internal temperatures, occupancy gains, lighting gains, equipment gains, and ventilation rates as set out in CIBSE Guide A and BB101.4 The ventilation heat recovery required to keep each modelled room variant was heat balanced, or in ‘thermal equilibrium’ – that is, the room heat gains minus the fabric and infiltration heat losses were balanced by the temperature of the ventilation air being supplied by the MVHR. This was calculated using steady state equations for an external temperature range of -5oC to +10oC.
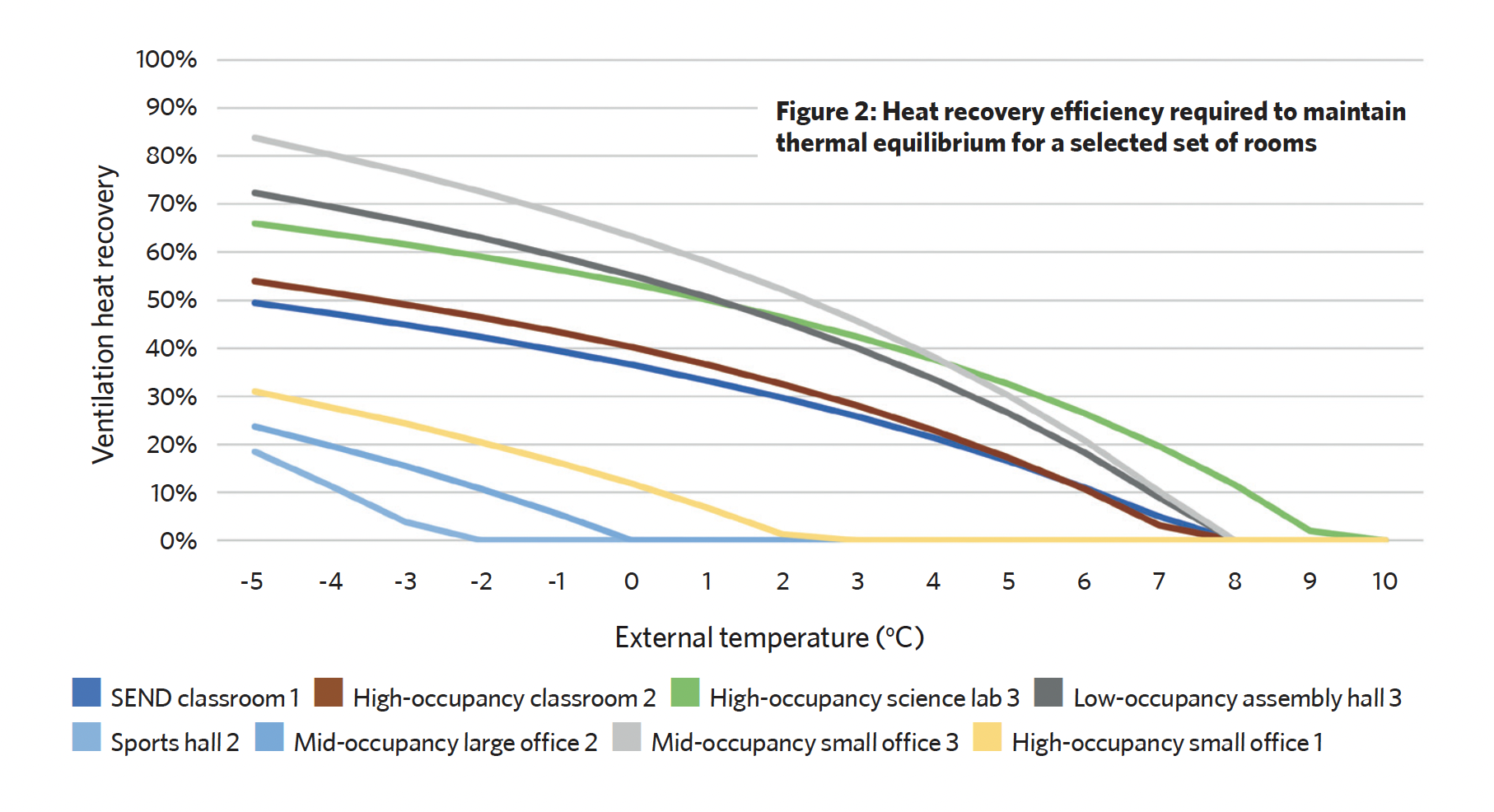
Figure 2 shows a selected set of the room results to indicate the range of ventilation heat recovery efficiencies required to keep the rooms in thermal equilibrium. This indicates that the optimum ventilation heat recovery efficiency varies greatly depending on the occupancy density, building construction and external temperature.
It is common that the effectiveness of ventilation systems’ heat exchangers are evaluated at an external temperature of -5oC, as that is one of the standardised test conditions. However, sizing the heat exchanger based on these extreme conditions will impact the efficiency of the systems at all other times. For example, referring to the future predicted weather files5 for south-eastern England, the external temperature is only expected at -5°C for one typically occupied hour (09:00 to 17:00) throughout the year. If -1oC is used as a representation of extreme external conditions, this would encompass 99.5% of occupied hours.
Figure 3 shows the results for the classrooms with an external temperature ranging from -1oC. The high-occupancy classroom with mid-range building thermal performance shows thermal equilibrium can be achieved with 42% heat recovery at -1oC external temperature. The model of the classrooms indicates that the required heat recovery efficiency increased as the occupancy density and building thermal performance decreased.
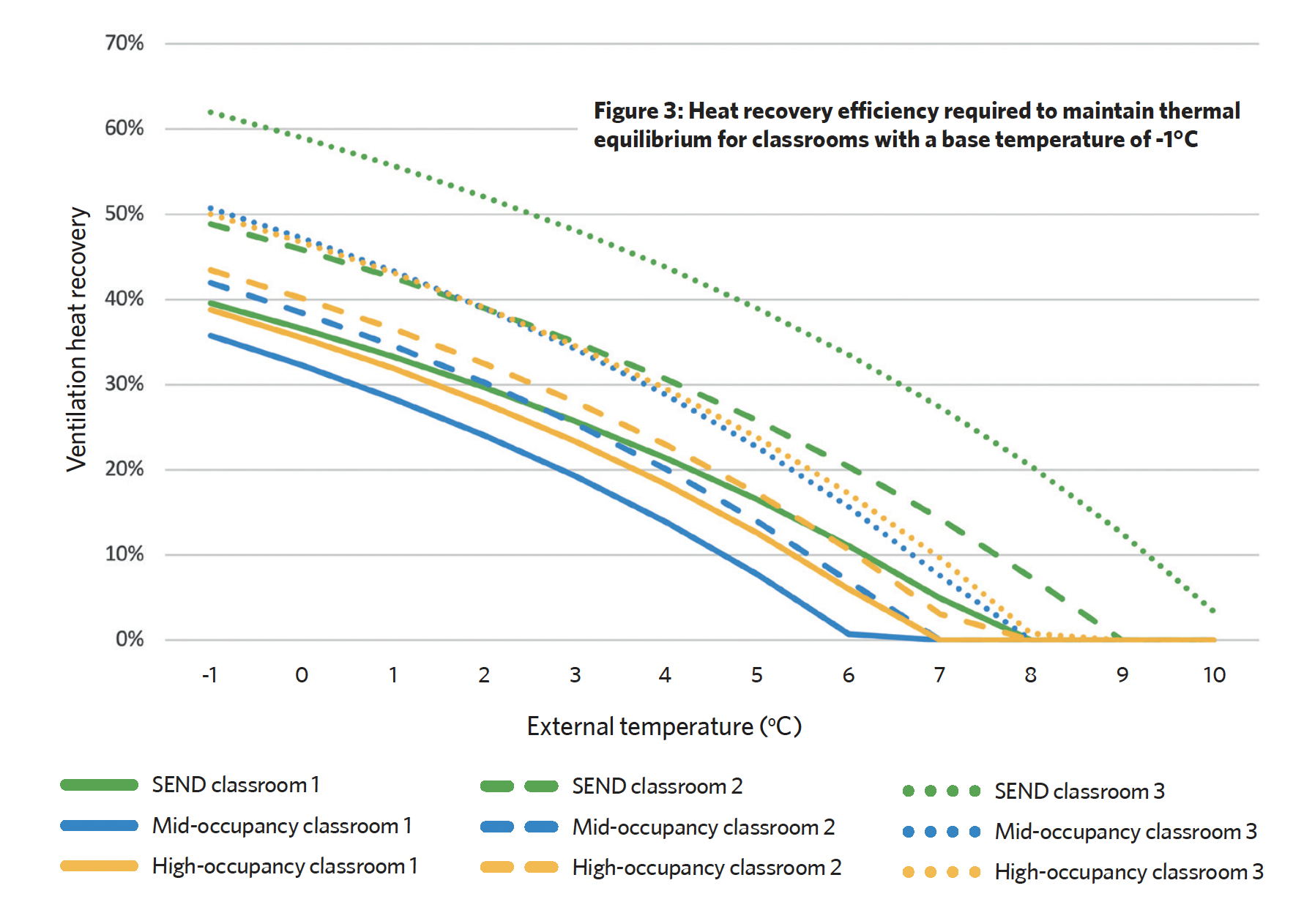
The same trend occurred for the science labs, although the required heat recovery efficiency was higher and differences more uniform owing to the ventilation rate being based on floor area rather than occupancy. The high-occupancy science lab with mid-range building thermal performance showed thermal equilibrium at 49% heat recovery at -1oC external temperature.
The results for the sports hall varied widely between general sport use and exam/high-occupancy use. While in sport use, and with an external temperature of -1oC, the heat losses resulting from ventilation and conduction are lower than the total heat gains in the room, hence no ventilation heat recovery is required. However, sports hall (exam) with mid-range building thermal performance requires 50% heat recovery.
The modelled output for the commercial offices showed the largest range of required heat recovery, owing to the building constructions ranging from well-insulated to fully glazed façades. The highly insulated variant showed no need for heat recovery, and all other constructions required less than 35% heat recovery. The small office and boardroom models required very little heat recovery where the construction was highly insulated, but in some cases required additional mechanical heat even if all the heat energy was recovered from the exhausted air. This was due to the very high ratio of (poorly insulated) façade area to occupancy density.
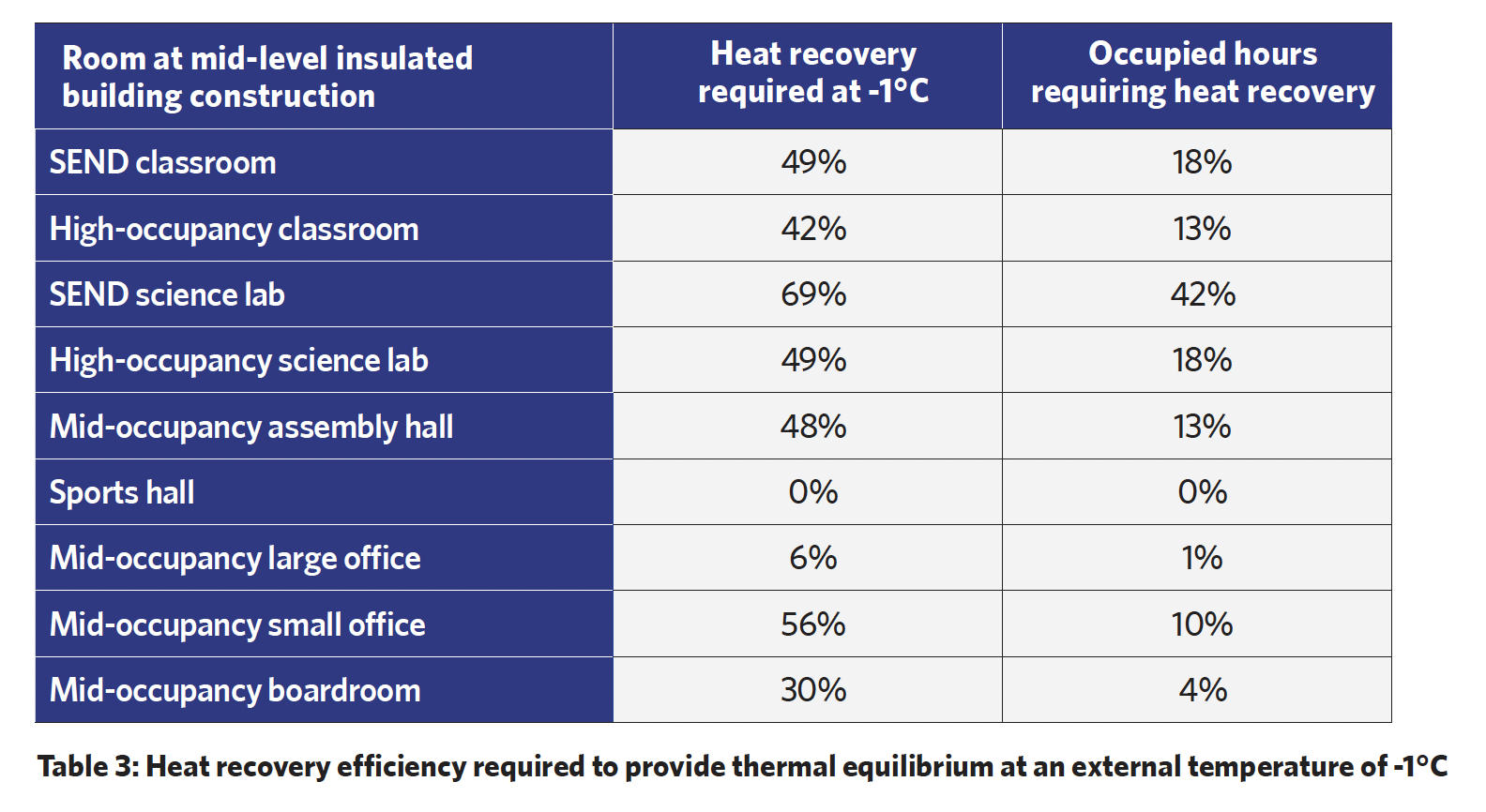
Table 3 summarises the heat recovery required to provide thermal equilibrium at an external temperature of -1oC for each of the modelled areas at typical occupancy and mid-level building construction, as well as the percentage of typically occupied hours where any amount of heat recovery is required. This indicates that the required ventilation heat recovery for most cases is less than that required in ErP legislation and, for many cases, heat recovery is only useful for under 20% of occupied hours.
The modelling applied in this study, and reported in this article, takes a simplified broad-brush approach in order to emphasise succinctly the need for further examination. This work suggests that it would be beneficial to consider ventilation systems with lower heat recovery efficiencies and high ventilation flowrate efficiency to reduce building energy use, rather than rely on prescriptive limiting values of heat exchanger thermal efficiency. As shown in Table 3, for much of the occupied period the HRS is not required, and so it is important that heat exchanger bypasses are designed with a low resistance to airflow and controlled robustly, since this path is likely to be used for the majority of the time that ventilation is required for typical buildings.
- With thanks to Kieran Kilgariff for the commentary and results from his study that have been lightly edited and reproduced in this article.
References:
- Commission Regulation (EU) No 1253/2014 of 7 July 2014 implementing Directive 2009/125/EC of the European Parliament and of the Council with regard to ecodesign requirements for ventilation units.
- CIBSE Guide A Environmental Design, CIBSE 2015.
- The Building Regulations 2010, AD L2, 2021 edition (2023 amends), HM Government, 202
- BB 101: Ventilation, thermal comfort and indoor air quality 2018, UK ESFA, 2018.
- Future weather file for London, Gatwick DSY 1 (moderately warm summer) for 2080, low emissions scenario, 50th percentile – www.cibse.org/weatherdata.