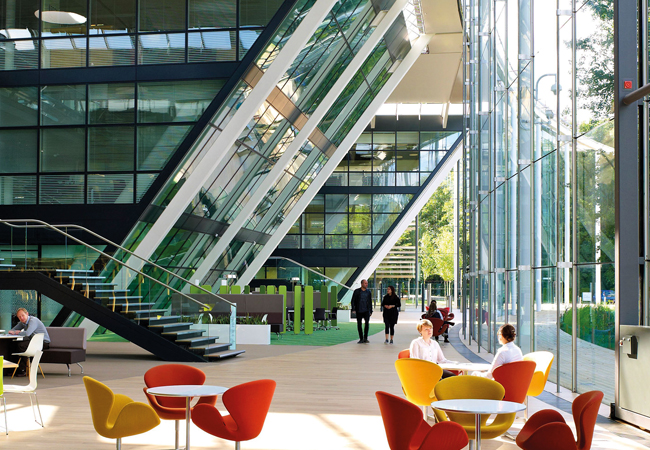
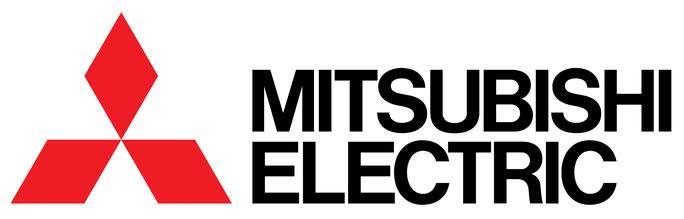
As governments and businesses around the world commit to net zero targets, whole life carbon (WLC) assessments are becoming increasingly important as a metric to help inform decisions in the construction and operation of built environments.
This CPD article will consider the concept of WLC, the relative impact of its constituents, and will reflect on current guidance that may be used to steer the building services community towards systems that have a more carefully considered impact of the future environment.
Whole life carbon (WLC) is a measure of the total carbon emissions associated with a building over its entire life-cycle, from the extraction of raw materials through to the demolition and disposal of the building. WLC is increasingly being used to assess the environmental impact of buildings and to inform decisions about design, construction, and operation. The components of WLC are embodied carbon and operational carbon.
Embodied carbon is that emitted during the construction of a building – including the extraction, processing, and transportation of materials; the construction process, which includes the fabrication of components, transport and construction; and the post-hand-over maintenance, repair and replacement, demolition, dismantling and ultimate disposal of the building materials.
Operational carbon is the carbon emitted during the operation of a building, such as the direct and indirect emissions resulting from the energy consumed by heating, cooling, lighting, and the use of appliances. In building services applications, there is likely to be a complex relationship between the embodied and operational components, which will be influenced by the performance of the thermal envelope, occupancy patterns and building use, the loads in the space, and the design of components, systems and sub-systems.
WLC assessments provide a tool to benchmark and minimise a building’s environmental impact across the entire life-cycle of a building – and they can help to improve the value of buildings, by demonstrating that efforts have been made to reduce its environmental impact while also complying with corporate social responsibility (CSR) goals, which include reducing environmental impact.
As highlighted by the 2017 RICS Professional statement on whole life carbon assessment for the built environment,1 embodied carbon can make a larger contribution than that of the operational carbon across the building’s life when considering the whole building. The RICS document includes an illustration of the constituents of modelled whole life carbon over a 60-year building life-cycle for several examples including those for a London speculative office development, as shown in Figure 1.
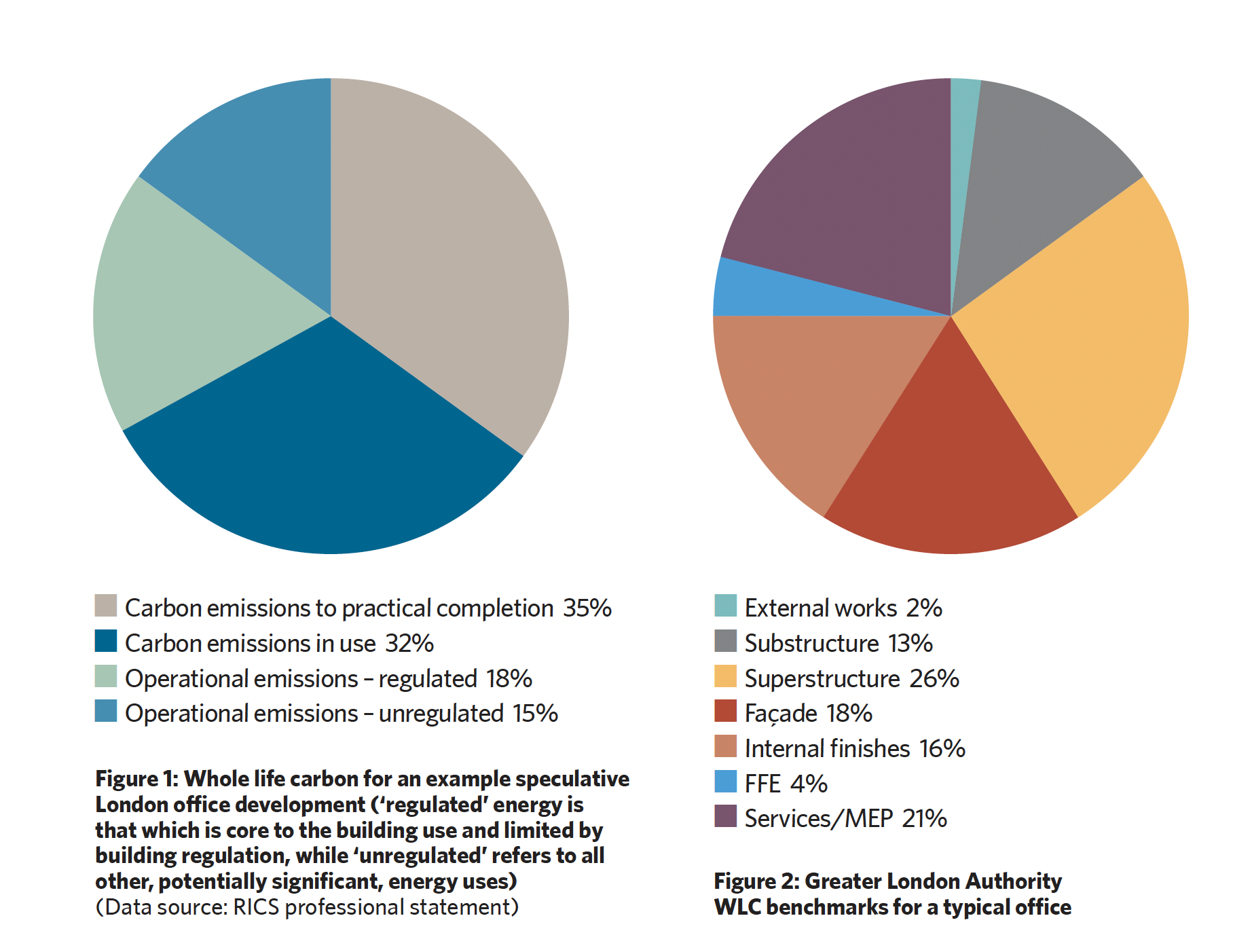
When considering the whole building, as reflected in this data, the embodied carbon accounts for a significant proportion – approximately 70% – of the total over a building’s lifetime, and undoubtedly is an important consideration when assessing the design options for fabric and systems.
However, it is inappropriate to draw the conclusion that embodied energy is so dominant that it eclipses the need for careful consideration of operational performance of specific elements, particularly those designed by the building services engineering (BSE) sector (which encompasses sub disciplines including those often referred to as mechanical, electrical and public health (MEP), and heating ventilating, air conditioning and refrigeration (HVAC&R) – as well as the optimisation of the hygro-thermal performance of building envelope and structure).
As illustrated in the example in the boxout ‘Simplified whole life carbon assessment’, the values of embodied carbon in the example heat pump and gas condensing boiler application are swamped by the operational carbon in less than a year of operation.
Figure 5 illustrates the relative impact of embodied and operational carbon by illustrating the application of a condensing gas boiler and an air source heat pump for an office type development that has a peak space heating load of approximately 400kW, and an approximate annual space heating requirement of 800,000kWh per annum. This is based on the following basic calculations where the heating flow water temperature is assumed as 55°C for this simplified example. A modular gas boiler delivering circa 400kW of capacity with an efficiency of 90%, has a mass of approximately 500kg. Using the CIBSE TM65 average data sets for embodied energy, An air source heat pump installation capable of delivering circa 400kW heat capacity (based on five modular heat pumps similar to that shown in Figure 4) with a SCOP of 3.43 at 55°C flow temperature. The manufacturer supplied assessment12 employed a CIBSE TM65 mid-level calculation to determine the embodied carbon as 13,915kgCO2e per module, resulting in a total of 69,575kgCO2e = 70 tonnes embodied carbon for five units. Heat pump with SCOP 3.43 = 800,000/3.43 =233,236kWh electrical consumption per annum at 0.19338kgCO2e.kWh-1 (BEIS June 2022) = 45 tonnes CO2e per annum.Simplified whole life carbon assessment
7kgCO2e.kg-1 for a gas boiler. So, 500kg x 7 = 3,500kg = 3.5 tonnes CO2e embodied carbon for the gas boiler. 90% efficient condensing gas boiler = 800,000/0.9 =888,889kWh gas consumption per annum at 0.18254kgCO2e.kWh-1 (BEIS June 2022) = 162 tonnes CO2e per annum
This does not negate the need to understand the embodied energy but emphasises the requirement to carefully assess the WLC when comparing solutions. For example, an increase in a manufactured product’s embodied carbon (for instance, by using a more effective but heavier heat exchanger or more complex and extensive controls) may improve its operating performance (by even a relatively small amount) so that over the building’s life-cycle there is a significant reduction in carbon emissions.
The BSE design process has historically developed to minimise the environmental impact, as well as cost, of installed systems through optimising the operational aspects. BSE operational performance may be assessed and predicted by employing one of the many mature modelling tools. As noted2 by Dougherty in the UK, the ‘best methodology we currently have for calculating operational energy is set out in CIBSE Technical Memorandum 54 (TM54)3 … based on the intended use and operation of the building’.
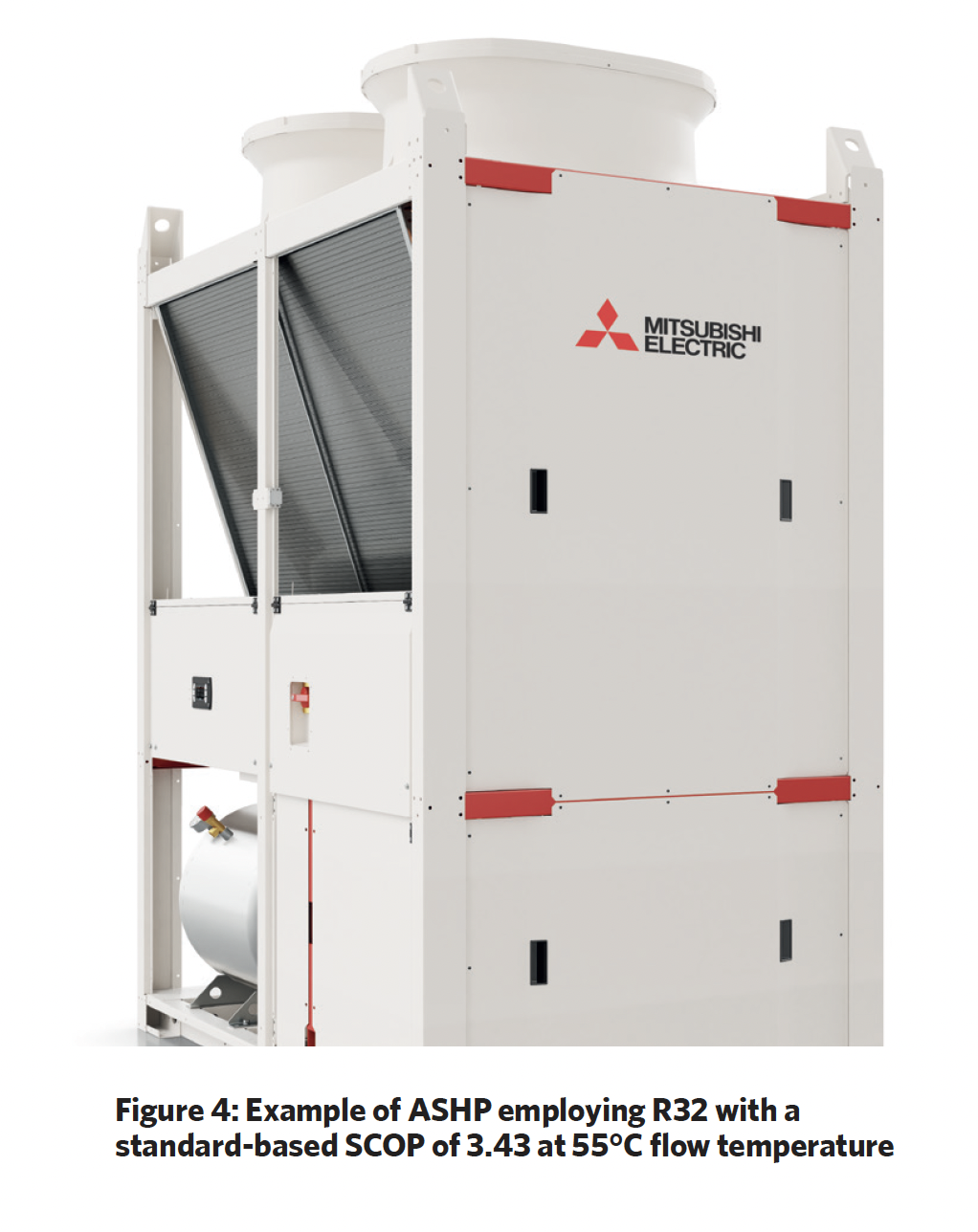
An indication of the impact of BSE that might be expected on the whole building’s life-cycle is provided by the London Plan Guidance,4 where the benchmark for offices sets BSE as being responsible for 21% of WLC, as shown in Figure 2.
As noted by the recent UK House of Commons report,5 the Building Regulations do not address embodied carbon emissions from buildings or the embodied carbon costs of actions to reduce operational energy use. However, it observes that the ‘primary gap preventing consistent assessment across the sector is not a lack of underlying standards or guidance but the lack of prescriptive assessment boundaries and defaults that are typically prescribed within a national methodology’.
A calculation method to assess the whole life environmental performance of buildings is described by British Standard BS EN 15978:2011.6 The standard breaks down the life-cycle of a building into life-cycle modules, as shown in Figure 3, but its detail is not sufficiently precise or informative to undertake a clear WLC assessment.
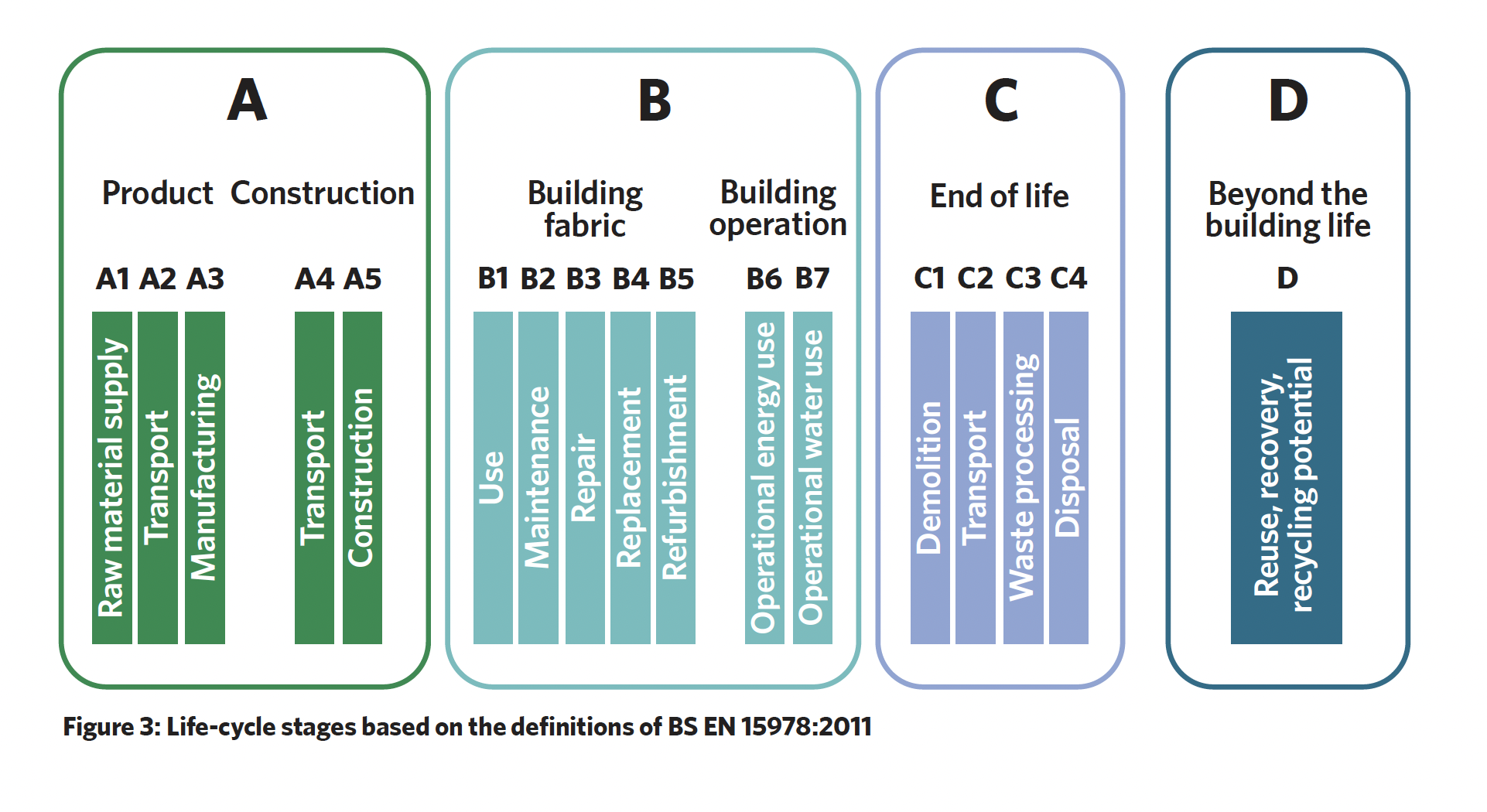
The Royal Institution of Chartered Surveyors (RICS) 2017 Professional Statement on Whole Life Carbon was developed in response to the inconsistent application of BS EN 15978 in the UK, and aims to provide principles and practical guidance for whole life carbon assessment to be adopted across UK industry. Alongside the Whole Life Carbon Network (WLCN) and LETI (Low Energy Transformation Initiative), who have developed a methodology for calculating whole life carbon, CIBSE broadly supports the RICS methodology.
Despite the general acceptance of the RICS framework, last year’s RICS sustainability survey7 points to a lack of industry engagement in the measurement of embodied carbon, with half of the global respondents reflecting that there are either no assessments being undertaken or, if they were, they had minimal impact on the selection of materials and components.
Many respondents agreed that it was the lack of established standards, tools, databases, benchmarks and guidance that was preventing greater application of WLC assessments. Currently, there is no globally standardised methodology for calculating embodied carbon for products, components and building elements. However, some manufacturers do produce environmental product declarations (EPDs), as described in BS EN 15804,8 for their products.
These provide information on the embodied carbon of a product, as well as other environmental impacts – such as ozone emissions, acidification, water use and waste generation. For MEP products and sub-systems where EPDs are not available, CIBSE TM659 provides guidance on how to measure and report embodied carbon. This includes two alternative approaches – a ‘basic’ calculation method requires less information and includes a scale-up factor to cover further life-cycle stages; and a ‘mid-level’ calculation method requires more information, thus is more comprehensive, but is still not as robust as the information available in an EPD.
TM65 emphasises the importance of ‘lean’ MEP, where the building is designed and constructed to reduce the need for services such as heating and cooling. A large proportion of embodied carbon from MEP products is associated with the product stage (as in Figure 3) owing to the majority of MEP components being made of metals, electronics and plastics, as well as their complex supply chains. Bagenal George et al provide extensive worked examples (in their freely downloadable paper10) that investigates the WLC of four types of heat-generation equipment by applying the methodologies of TM65: gas boiler; gas-fired combined heat and power (CHP); air source heat pump (ASHP); and variable refrigerant flow systems (VRF).
Among other findings, their work concurs with TM65 that for those systems employing refrigerant cycles the WLC is strongly influenced by the global warming potential (GWP) of the refrigerant and, in some situations, refrigerant leakage has a higher impact than operational carbon emissions. As such, if refrigerants with a relatively low GWP are employed, this can be significantly reduced.
The UK Parliament POSTbrief 4411 includes a useful commentary on practical strategies to reduce the whole life carbon impact of buildings, which emphasises the importance of exploring opportunities to reuse, repurpose and refurbish existing buildings and systems to avoid the need for the construction of new built systems.
Principles that are particularly pertinent to BSE include optimising the use of materials and products and their associated whole life emissions – this requires a holistic approach, since improving the operational efficiency of a building may be directly achieved by, for example, installing energy-efficient equipment and systems (including systems for renewable energy) or, more obliquely, by improving the building’s fabric performance or operational management, so that the heating, cooling, and electrical loads are minimised. It highlights the benefit of assessing the embodied carbon of materials and products for maintenance purposes (and potential retrofit) and their potential to reduce operational carbon.
The principles of the circular economy should be maintained, regardless of the route that is followed. This includes considering the reuse, recovery, recycling, and prospective recovery of embodied energy from materials. While this ‘stage D’ activity may be easier to theorise about than to practically complete at the moment, it is important to continue to explore and develop the methods and techniques in order to better understand the complete whole life carbon of a building.
As concluded by the House of Commons Environmental Audit Committee,5 there is no UK government policy requiring the assessment or control of embodied carbon emissions from buildings. As a result, no progress has been made in reducing these emissions within the built environment.
However, there is a broad cross-section of the construction industry willing and able to undertake whole life carbon assessments, and in the absence of an approved UK national methodology, the committee suggests that the RICS Professional Statement on WLC is used as the accepted industry methodology. In any case, building professionals will increasingly need to consider the whole life carbon emissions of their designs in order to meet the needs of clients, buildings and society.
This is a challenge, but through employing the growing range of lower-carbon and energy-efficient equipment, professionals will need to seize opportunities to deliver a lower-carbon future.