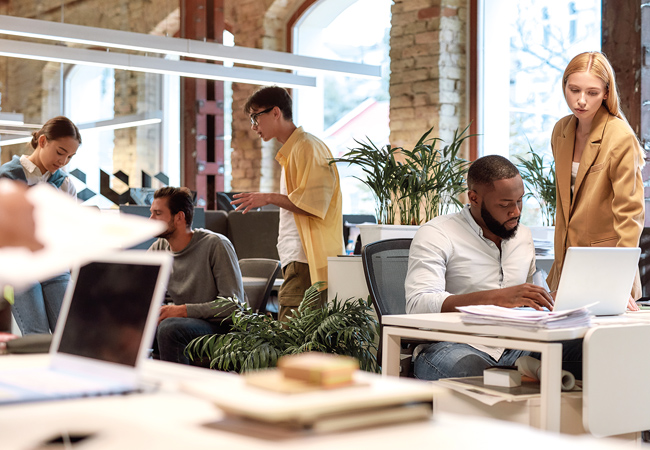
This CPD article will consider the recent evolution in the understanding of occupant thermal comfort and how this has excited the quest for personal environmental control.
Traditionally, prior to the advent of centralised environmental systems, individuals would have moderated their own comfort by adjusting local temperature (possibly both dry-bulb and radiant temperatures) and lighting, and potentially would have also influenced the local air quality, humidity, and the acoustic environment.
This may appear to be an extensive list, but in simple, traditional, non-air conditioned built environments, an individual may well have been able to influence many – if not all – of these by altering their clothing; controlling the local window openings, shading devices and curtaining (drapes); and, when satisfaction could not be achieved through these passive means, by employing local active environmental devices such as heaters, fans and lights. As buildings increased in size, and technology evolved to offer climate control solutions, environmental systems became more centralised, mainly for practical management and economic reasons.
Over the past half-century, there has been an explosion in studies undertaken to better understand what is required for occupants to be satisfied with their internal environment – in most cases, to create conditions that are conducive to maximum productivity. Ole Fanger’s seminal work from more than 50 years ago established the comfort equation and introduced the terms ‘predicted mean vote’ (PMV) and ‘percentage population dissatisfied’ (PPD), as well as the associated comfort diagrams that have since developed and been codified in BS EN ISO 77301 and other standards across the world.
The use of a broad-brush comfort model, such as that in BS EN ISO 7730, to set steady-state environmental conditions to deliver the most statistically acceptable uniform, indoor environment, is typically considered appropriate for the majority of building occupants whose attributes coincide with the values of the defining parameters used to evaluate the PMV. However, occupants are increasingly disparate in their choice of clothing and activity levels, let alone their physiology, physical condition and mental state.
For example, the relaxation in dress codes in both white- and blue-collar occupations, as well as changes in general sartorial etiquette, will mean that in many indoor spaces the range of ‘clo’ values (as used by PMV to define the insulation effect of clothing) will be practically unpredictable and extremely variable. The influence of the built environment on occupant health and wellbeing has been well established (as discussed in CIBSE TM52 Health and wellbeing in building service) so, alongside the demands of productivity, there are compelling drivers to facilitate environments and controls that improve occupant satisfaction.
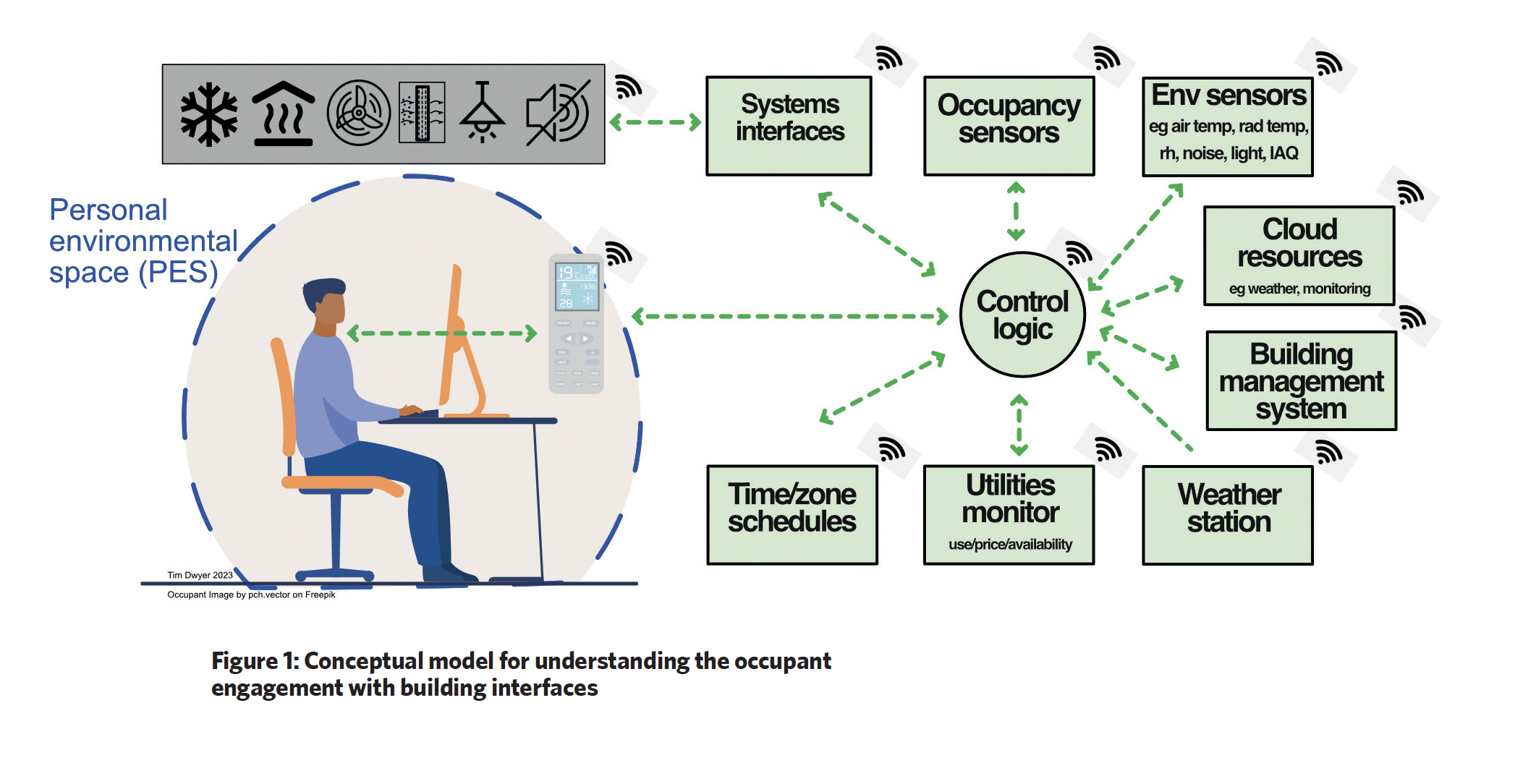
The idea of adaptive comfort evolved and was formalised by Humphreys and Nicol2 around the turn of the century, so combining behavioural, psychological and physiological factors to provide a more realistic insight into an individual’s opportunity to control personal comfort.
Restoring some authority to the individual underpins the concept of ‘adaptive control’3 that is widely applied, particularly in naturally conditioned buildings. This recognises that a person’s expectations of comfort conditions – and so the environmental setpoints – relate to a wider range of parameters, notably including recent trends in the external local climate and the opportunity for personal adaptation. TM52 suggests that designers should accommodate this by affording the occupant with some control over the environment (for example, varied seating locations, openable windows, or resetting a thermostat) and, ideally, flexibility in clothing.
Many research projects (as illustrated by the extensive literature review4 undertaken by Grassi et al) have improved the understanding of an individual’s contentment, comfort, and even happiness, with their immediate environment. In real buildings, occupants will be exposed to continuous variations in their local environment. These perturbations in conditions have been shown to impact an occupant’s overall sensual pleasure, which is often related in terms of ‘hedonism’, the attempt to maximise pleasure and minimise pain.
To describe the circumstances through which a stimulus (or variation) can induce either a pleasant or unpleasant experience, depending on the person’s internal body state, Michael Cabanac5 originated the word ‘alliesthesia’ in 1971 (contemporaneously with Fanger’s early work). Fanger had also noted6 that ‘climate monotony’ potentially led to ‘increased fatigue, lower arousal and lower performance’ but, at the time, although having undertaken preliminary studies, such claims had not been supported by experimental evidence.
As reported by André et al in their recent review paper,7 reflecting on the opinions of the highly cited ‘thermal comfort’ researcher Richard de Dear, in order to please users, it is important to avoid thermal boredom. Transitory conditions created by occasional stimuli allow the production of positive alliesthesia – a kind of relief generated by the body returning to its point of equilibrium after an extreme thermal sensation, which is identified as thermal pleasure. The further away from the point of equilibrium the body is, the greater the pleasure generated when there is a return to equilibrium; that is, the greater the variation, potentially the greater the thermal pleasure generated.
He et al describe8 alliesthesia in terms of ‘thermal pleasantness’ that accompanies the correction of thermal imbalances across the body. Simple examples would be a person moving to stand in front of a roaring fire in an otherwise cold room, or to switch on a desk fan to increase cooling for the face and neck in a warm environment. André et al explain that temporal alliesthesia is somewhat limited, since overcompensation to provide thermal pleasure is short-lived and certain combinations of thermal sensations from body parts can exert a more pleasurable sensation than that of uniform whole-body comfort. However, perceptions from individual body parts experiencing strongest discomfort (such as overcooled feet) can also dominate.
In his 2020 paper,9 Cabanac revisited the definition of alliesthesia and, by referring to multiple intervening published studies, identified parameters that were thought to contribute to alliesthesia. Aside from traditional thermal comfort factors, this encyclopaedic list included smell, taste, pain, exercise, scholastic performance, appreciation of ‘art’, sexual arousal, plus mental processes including curiosity, appreciation of money, decision making, grammar, ethical consideration and aggression, as well as politics, learning, and memory. His new definition is suitably all-embracing.
It would be impossible to measure, let alone account for, many of these potentially changing parameters when determining appropriate responses from an environmental control system. However, they will likely all play a part, to a greater of lesser extent, in the holistic satisfaction of occupants. The challenge is to obtain meaningful feedback from occupants and so directly or indirectly provide opportunity for them to exert influence on the environmental systems.
There has been much work in this area over many years, both in terms of the direct and indirect environmental adaptation, employing various interfaces with, for example, windows, window shades/blinds, thermostats, and lighting controls, as reported in the recent review10 by Day et al; and by employing digital sensors and interfaces enabled by increasingly ubiquitous wireless connectivity and the Internet of things (IoT), as illustrated in the concept of Figure 1.
Maintaining connectivity for comfort
Connectivity is the crucial element that enables the context sensitive-operation of environmental systems to meet the demands of individual occupants. Wireless networks provide flexibility that, practically, can allow individual users and devices to connect with a control system, particularly where it is difficult or impossible to run traditional wired networks. Ultimately, as well as helping to deliver suitable environmental control, such connectivity provides a route to optimising the use of equipment that consumes the most energy, such as fans and chillers.
Mesh wireless networks are an increasingly popular choice, as they provide redundancy and are effectively ‘self-healing’, as each node (which might, for example, be a temperature-sensing node, or a personal feedback device) in the network is connected to at least two other nodes and can still function, with data routed through other nodes, even if some nodes are down. This allows for a more reliable and robust network than a traditional star wireless topology, where each node is connected to a central hub.
Mesh networks are readily scalable, so they can be easily expanded to accommodate more devices or users and do not require as much cabling or infrastructure as traditional networks. This approach can reduce the time needed to install, set up and commission the system, as well as easing system reconfiguration when changes are made to room layouts or usage patterns. Such networks will support encrypted networking protocols that enhance security against cyber threats.
To enable interoperability with a wide range of manufacturers’ equipment, the chosen networking environment should ideally be capable of connecting to other systems through commonly used, robust control protocols, such as ASHRAE’s BACnet. This allows connection to a variety of applications and sub-systems including mobile and cloud-hosted devices, front-end computers, general-purpose direct digital controllers, and application-specific or unitary controllers.
It is widely considered that when people are able to control their own environment, they are more likely to be comfortable and so more productive (as summarised by various research papers reviewed by Al Horr et al in section 3 of their 2017 paper ).11 It has long been appreciated, and noted by Leaman and Bordass,12 (when considering office occupants) that the inability to freely adjust building controls and the failure of the associated control systems to produce desired effects can create enduring discomfort and frustration. They note that ‘people who have control are also less critical of actual conditions, as in naturally-ventilated, “freerunning” buildings.
In air-conditioned buildings, lower levels of personal control seem to make people less tolerant, even though conditions may be objectively better.’ Various terms have been coined, including ‘personal environmental control’ (PEC), and the more wide-ranging ‘personalised environmental control systems’ (PECS) and ‘personal comfort systems (PCS)’ that are used to describe the ability of individuals to control their own local environment both directly through active interventions possibly employing local or personal environmental devices; and/or indirectly, with personal sensing and control devices.
Leaman and Bordass noted that as ‘perceived control is also linked to satisfaction and low incidence of chronic illness, and it is reasonable to surmise that control, comfort, satisfaction and productivity are all positively associated.’ They do however caution that the perception of control and actual control may not be the same thing, especially if conflicts or control complexities are unwittingly introduced that make the building systems more challenging to maintain and manage. Although the principles of comfort have clearly been increasingly well understood, the consequences of poor environments appear to prevail.
As recently reported13 by Graham et al, based on a 20-year longitudinal set of US office surveys, a third of occupants reported that temperature has the largest negative impact on self-reported productivity (second only to acoustical quality at 39%). In their 2022 commentary,14 Arens and Zhang of the US-based Center for the Built Environment (CBE), reflecting on a paper15 by Parkinson et al, note that buildings are routinely overcooled, particularly in summer, when 76% of complaints of cold temperatures came from women.
They highlight that ‘decentralising thermal control to the occupant level addresses two of the current building industries’ greatest deficiencies: the universally high rate of occupant dissatisfaction with commercial building thermal environments and the more than 20% of total global energy consumption and CO2 generation that currently go into producing those remarkably unsuccessful indoor environments’. In extolling others to share experiences, they explain that PCS ‘can deliver a decentralised building thermal control, in which occupants control their local environments with personal devices while the amount of central space conditioning is scaled back’.
Thermal systems that heat and cool individuals without affecting the environments of surrounding occupants, which are under the individual’s control, tend to be devices locally positioned on, or incorporated in, workstation furniture such as chairs, desktops, or positioned near the feet and legs, such as those illustrated in Figure 2. Such systems may provide opportunities to reduce the total costs of central environmental systems that are used to provide a tempered global internal environment, while allowing local, personal devices to maintain comfort as well as improve health and wellbeing.
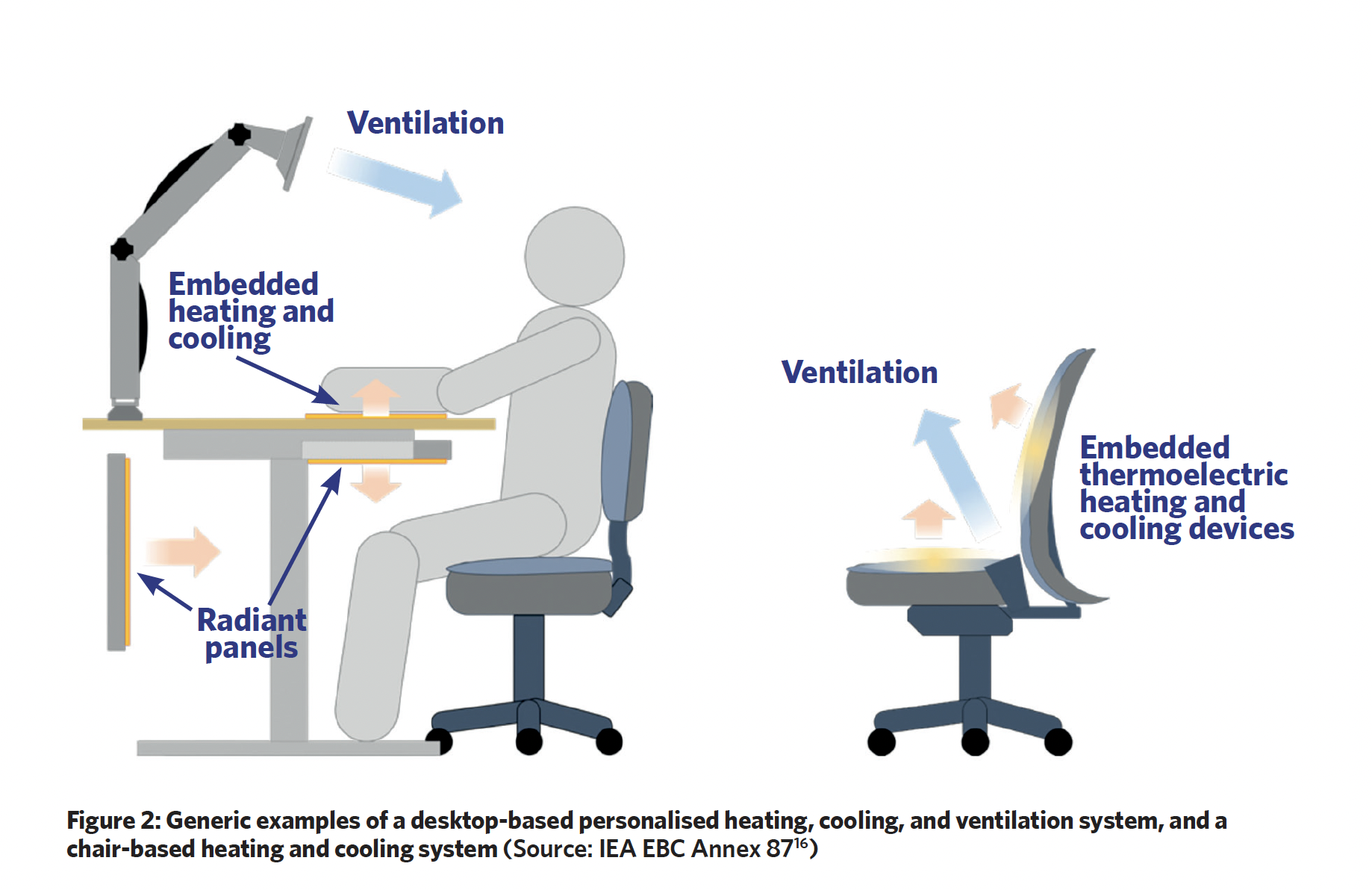
The recently convened International Energy Agency (IEA) Energy in Buildings and Communities Programme project, annex 8716 Personalised Environmental Control Systems (PECS), aims to disseminate knowledge on design and use of PECS. The introduction to the annex’s work reasserts that, as well as substantially improving personal comfort, health and energy efficiency of the entire heating, ventilation and air-conditioning system, personalised ventilation will also protect against cross contaminations, which are critical in open-plan offices and workplaces where occupants work in close proximity. They consider that there will be an increasing interest and market for PECS as buildings need to be pandemic-proofed.
As noted by Arens and Zhang, there is still development required to coordinate, in real time, the devices’ sensing and communicating capabilities with buildings’ environmental control systems. However, although the transition to decentralised environmental systems and personal control may be a challenging prospect, advances in connectivity – as well as new generations of sensing and feedback technology – together with increasing interest from building users and manufacturers, promise to open the highway towards personal environmental control.
References:
- BS EN ISO 7730:2005 Ergonomics of the thermal environment. Analytical determination and interpretation of thermal comfort using calculation of the PMV and PPD indices and local thermal comfort criteria, BSI 2006.
- Humphreys, MA and Nicol, JF, Understanding the adaptive approach to thermal comfort, ASHRAE Winter Conference 1998.
- bit.ly/CJNov22CPD32 – accessed 10 May 2023.
- Grassi, B, A Review of Recent Literature on Systems and Methods for the Control of Thermal Comfort in Buildings, Applied Sciences, 2022, bit.ly/CJJun23CPD21.
- Cabanac, M, Physiological Role of Pleasure: A stimulus can feel pleasant or
unpleasant depending upon its usefulness as determined by internal signals, Science, 1971, bit.ly/CJJun23CPD22. - Fanger, PO, Assessment of Man’s Thermal Comfort in Practice, British Journal of Industrial Medicine, 1973, bit.ly/CJJun23CPD23.
- André, M, User-centered environmental control: a review of current findings on personal conditioning systems and personal comfort models, Energy and Buildings, 2020, bit.ly/CJJun23CPD24.
- He, Y et al, Creating alliesthesia in cool environments using personal comfort systems, Building and Environment 209, 2022.
- Cabanac, M et al, Alliesthesia. Up-date of the Word and Concept, AJBSR 2020,
bit.ly/CJJun23CPD25. - Day, JK et al, ‘A review of select human-building interfaces and their relationship to human behavior, energy use and occupant comfort, Building and Environment 2020, bit.ly/CJJun23CPD26.
- Al Horr, H et al, Occupant productivity and indoor environment quality: A case of GSAS, International Journal of Sustainable Built Environment, 2017, bit.ly/CJJun23CPD27.
- Leaman, A and Bordass, W, Building Design, Complexity and Manageability, Facilities, 1993, bit.ly/CJJun23CPD28.
- Graham, LT et al, Lessons learned from 20 years of CBE’s occupant surveys, Buildings and Cities, 2021 bit.ly/CJJun23CPD29
- Mainstreaming Personal Comfort Systems (PCS), CBE, 2022, bit.ly/CJJun23CPD210.
- Parkinson T et al, Overcooling of offices reveals gender inequity in thermal comfort, Scientific Reports, 2021, bit.ly/CJJun23CPD211.
- bit.ly/CJJun23CPD212 – accessed 10 May 2023.