However, in many situations, as cooling requirements rise, this may not be sufficient. At the same time, the airtightness standards for modern housing provide good-quality envelope design that do not provide the sometimes-fortuitous ventilation effect arising from the infiltration in the leakier buildings of the past. So, as reported in a recent EU publication,1 older properties that are refurbished with modern standards of thermal insulation and improved fabric airtightness will need to ensure that the upgraded space is supplied with adequate ventilation.
The recently introduced Building Regulations for England Approved Document part O1 Overheating Mitigation of the Building requires that dwellings should include reasonable provision to limit unwanted solar gains in summer and, where that is not sufficient to maintain acceptable temperatures, to provide an adequate means to remove heat from the indoor environment.
Adaptive comfort
The adaptive comfort model sets the required indoor temperature by current (and recent past) measurements of outdoor temperature. CIBSE Guide A explains that, as well as the preferred internal temperature depending on the occupants’ state, it will be influenced by the type of building (naturally ventilated; mixed mode; or air conditioned) and the occupants’ opportunity to employ operable windows and blinds, as well as a means of generating local air movement (other than the window).
As reported7 by the US Center for the Built Environment (CBE), people accept and even prefer a wider range of temperatures than is suggested by the PMV (deterministic) model. This difference is explained by psychological and behavioural adaptation. The recently published TM68: 2022 Monitoring indoor environmental quality notes that the adaptive model is widely used, including in BS EN 16798-1,8 CIBSE TM52 and TM 59 and ANSI/ASHRAE Standard 55.
A potential solution – for new or refurbished residences – is to employ a unitised, packaged balanced ventilation system, with total (sensible and latent) plate heat exchanger, which can be combined with an air-to-air heat pump to provide both ventilation air as well as heating and cooling of the incoming air.
The system continuously monitors the temperatures of outdoor and indoor air (estimated by extract sensors), so that the operational mode for the system may be optimised to provide the desired indoor temperatures. Operation is based on an adaptive temperature profile (see boxout ‘Adaptive comfort’) that takes account of the prevailing mean outdoor temperature – as well as occupant preferences – to provide a desired system set-point temperature.
Field tests were undertaken in houses in Luxembourg and in Modena, Italy, to establish how well this system could meet the comfort requirements of the occupants. (The installed mechanical ventilation with heat recovery unit for the Modena house is shown in Figure 1.) The thermal performance of the systems have been more fully reported2 by Bart Cremers, and his report has been abstracted to provide the basis of the discussion of the installations described in this article.
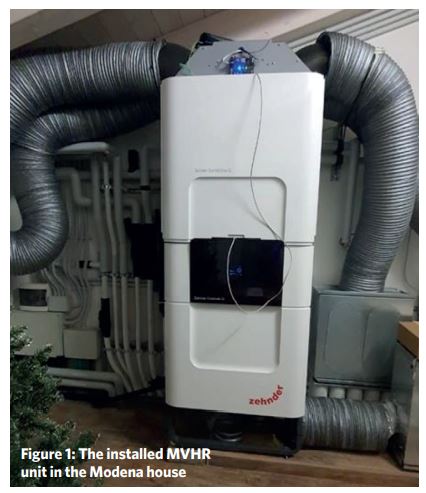
In a real application, such as a normally occupied house, it is challenging to determine a set of measurements that are representative of a standard comfort index (see the boxout ‘Assessing thermal comfort when avoiding overheating’). The tests reported in this article employed air temperature sensors (outdoor; supply after recovery; supply after conditioning; extract; and exhaust), humidities (outdoor; supply after recovery; extract; and exhaust), measurements of both the supply and extract air streams (flowrate; fan speed; fan duty), and electrical consumption.
All parameters were recorded over one year (18 July 2021 to 17 July 2022) every five minutes and summarised in hourly averages for further analysis.
Assessing thermal comfort when avoiding overheating
CIBSE TM 524 The limits of thermal comfort: avoiding overheating in European buildings provides guidance on assessing the maximum temperature that would be acceptable for thermal comfort where mechanical conditioning is employed. TM 52 acknowledges the practical challenges of continuously monitoring the six constituent variables of the predicted mean vote (PMV) with suffcient accuracy. Therefore, it recommends that the procedure for testing for overheating in mechanically-conditioned buildings is to monitor the operative temperature regularly in a number of representative spaces in the building.
Assuming occupants’ clothing has an insulation value of 0.5 CLO in summer, the recommended maximum temperature is 26°C. CIBSE TM 595 clarifies that ‘all occupied rooms should not exceed an operative temperature of 26˚C for more than 3% of the annual occupied hours’. This provides a proxy for a PMV of less than +0.5 (that is, the top end of the PMV band that is deemed comfortable by BS EN 15251:20076).
The Modena house, in a humid subtropical climate zone, was built in 2017 and consists of three floors with total floor area of 270m2. The insulation is within passive house limits, with a U-value of 0.132W.m-2.K-1 for the walls and 0.123W.m-2.K-1 for the roof. There are no other heating or cooling units used, other than the technology described in this article.
The house in Luxembourg, an oceanic climate that is typically 1K to 2K cooler than London, was built in 1981 and renovated in 2020, with a total floor area of 185m2. Typical U-values for this house are 0.14W.m-2.K-1 for the walls and 0.20W.m-2.K-1 for the roof. No other heating systems are used aside from the system under test.
A recent review3 undertaken by Spiekman et al has indicated that occupants have been shown to find it challenging to determine the optimal operation mode. In the normal operation of these systems, the various operational modes are automatically chosen by the control algorithm, based on the actual and the desired conditions.
During the cooler periods of the year, the temperature of the incoming outdoor air is initially raised by the heat recovery (‘avoided heating’ in Figure 2). If the adaptive controller requires a higher temperature, the coil will act in heating mode (supplied from the air-to-air heat pump) and will further increase the temperature of the supply air (‘active heating’ in Figure 2).
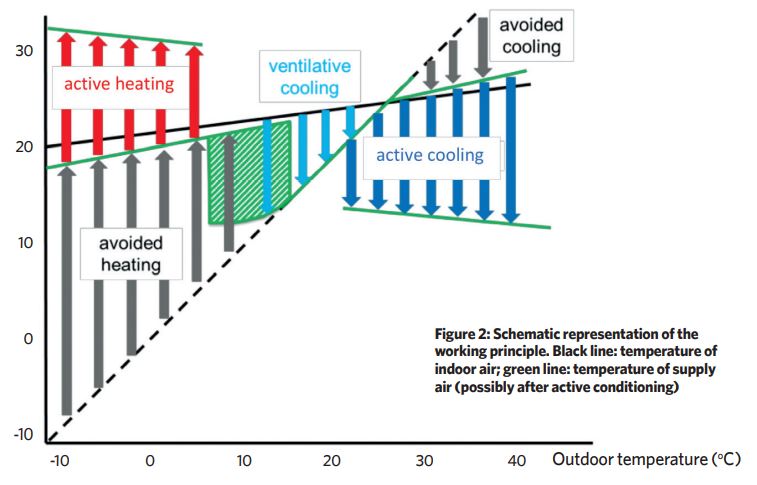
When temperatures outside are warmer than inside, the heat exchanger reduces the temperature of the incoming outdoor air to approach the cooler indoor air temperature (‘avoided cooling’ in Figure 2 ). If there is a need for further cooling, the coil (supplied from the air-to-air heat pump) will operate in cooling mode, providing both cooling and, potentially, dehumidification (‘active cooling’ in Figure 2).
During the periods of the year with mild temperatures, heat recovery automatically reduces as the temperatures inside and outside approach each other, or totally shuts off, with the adaptive controller activating a bypass in order to bring in cooler air. This period is shown as ‘ventilative cooling’ in Figure 2.
The cooling effect of the installation on the indoor climate in summer can be demonstrated by the example period shown in Figure 3 (in Modena). During the first five days, the ventilation system including recovery was operational, but the active cooling had been switched off.
The supply temperature during the day was brought to the indoor level (effect of cold recovery), but the extract temperature remained at a high level, with extract temperatures up to 30°C. During nights, the bypass was activated to bring in the cool outdoor air, with the resulting supply air temperature being close to the outdoor air temperature.
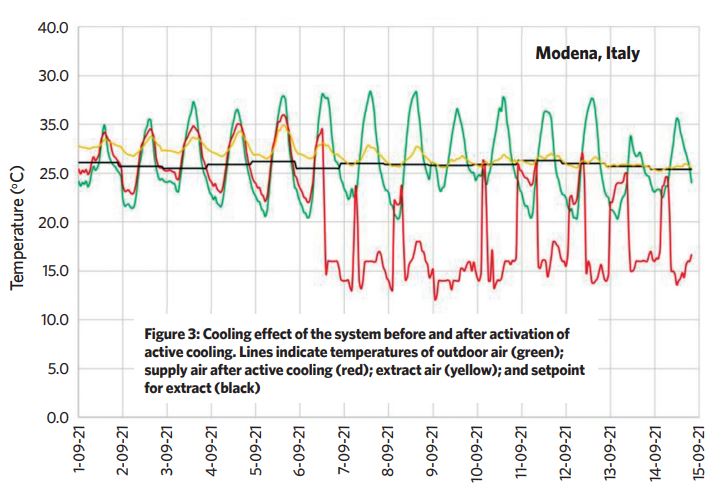
After five days (6 September 2021), the active cooling was put into use. The heat pump brought the supply air to approximately 15°C. The cooling effect on the indoor climate can be seen during the course of a couple of days, with a gradual decrease in extract temperatures. After four or five days, the extract temperature had reached the desired setpoint of 26°C and remained at that level.
Extract temperatures for Modena ranged from 22°C (winter) to 27°C (summer) and 20°C (winter) to 25°C (summer) for Luxembourg. In Italy, both active heating and active cooling had been used, while in Luxembourg active cooling was hardly needed.
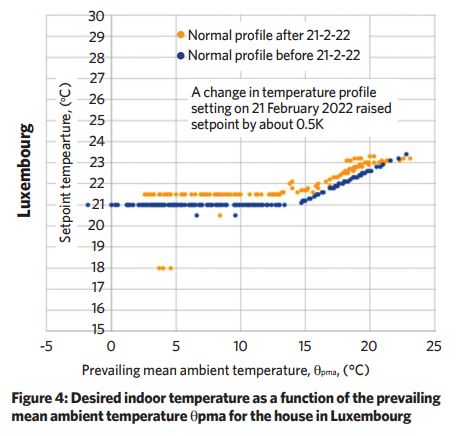
Figure 4 shows the desired indoor temperature related to the prevailing mean ambient temperature, θpma, as an example, for Luxembourg.
During the heating season (when θpma< 14°C) the setpoint is constant at 21°C. During the intermediate season, the setpoint rises, as the θpma increases, to 23°C.
In Italy, the typical flowrate was 300m3.h-1, but lower values of 1903.h-1 occurred during night settings and higher values up to 480m3.h-1 when there was a high requirement for active heating or cooling to condition the indoor climate. In Luxembourg, the typical flow rate was 200m3.h-1, but lower values of 80m3.h-1 were used when there was no occupation and higher values up to 300m3.h-1 when more heating or cooling was required.
Figure 5 shows the energy signatures for the systems that indicate the daily thermal energy output related to the daily averaged outdoor temperature. Active heating is displayed in red, and active cooling in blue. The differences between the Italian house and the house in Luxembourg are clear – in Luxembourg, active cooling is rarely needed, but active heating is used more frequently.
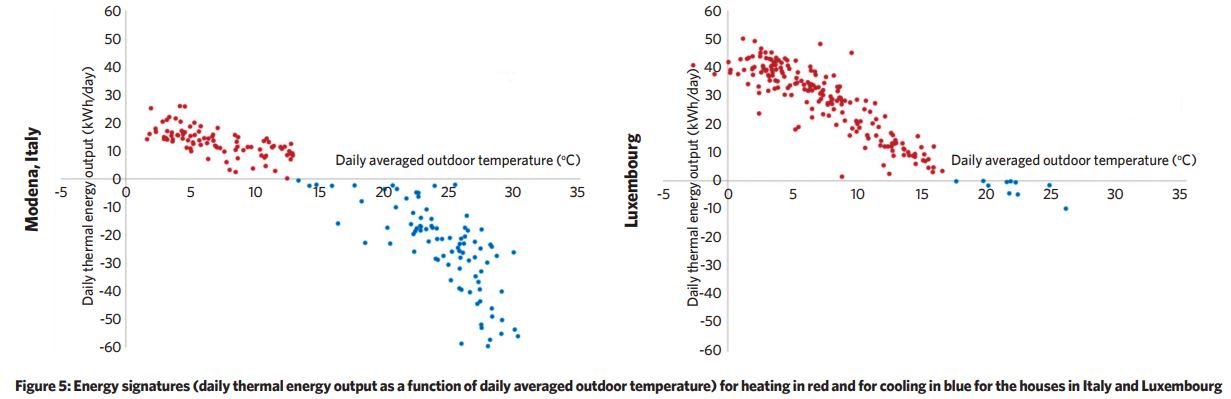
The actual values vary day to day, arising from a varying solar thermal gain, from occupation variation and from the chosen daily scenario. For example, the controller provides a selectable daily scenario to reduce or block the heat pump during a part of the day – for instance, at night.
The Italian house also shows a pronounced cooling season where the thermal cooling output is likely to include components of both sensible and latent cooling. For the Modena house, heating is required until outdoor temperature has reached a level of 13°C, whereas for Luxembourg it is 16°C.
It can take a couple of days for a very warm house to cool after the cooling has been switched on for the first time, and then the heat pump can maintain a comfortably cool environment. When outdoor temperatures are lower than indoor temperatures (for example at night), the heat recovery bypass activates to use simple ventilative cooling, which can be complemented, when needed, with active cooling from the heat pump.
The size of the heat pump in combination with the outdoor airflow rate decides the maximum thermal input that can be delivered to the house. In practice, the combination of a unitised MVHR system in conjunction with an air-to-air heat pump integrated by the adaptive controller, is potentially suitable for providing year-round filtered, outdoor air and active conditioning in nearly zero-energy buildings and those built to passive house standards. Variants of the system can, for example, include ground- or water-sourced cooling (direct or through using a heat pump), or local energy networks.
The use of automatic adaptive temperature control reduces unwanted heating and cooling, and the inclusion of the heat pump lowers heating energy consumption while providing the capability to prevent overheating.
© Tim Dwyer 2022.
References:
- Department for Business, Energy & Industrial Strategy, Heat and buildings strategy, October 2021. https://www.gov.uk/government/publications/heat-and-buildingsstrategy
- Department for Business, Energy & Industrial Strategy, August 2021, UK hydrogen Strategy. https://www.gov.uk/government/publications/uk-hydrogen-strategy
- Department for Levelling Up, Housing and Communities and Ministry of Housing, Communities & Local Government, Consultation outcome, The Future Buildings Standard, Dec 2021
- Sojoudi, A., Sefidan, A. M., & Alam, K. (2021). Chapter 7 – Hydrogen production via electrolysis:Mathematical modeling approach. Retrieved from Science Direct: https://doi.org.10.1016/B978-0-12-822525-7.00012-3
- Kayfeci,M., Kecebas, A., &Bayat, M. (2019). Solar Hydrogen Production, Retrieved from Science: https://doi.org/10.1016/b978-0-12-814853-2.0003-5
- Department for Business, Energy & Industrial Strategy, June 2022, Prices of fuels purchased by non-domestic consumers in the United Kingdom excluding/including CCL.
- Department for Business, Energy & IndustrialStrategy, Jan 2022, Conversion factors 2021: condensed set (for most users) – revised January 2022