Air in buildings is likely to contain contaminants, including particulate matter (some biological in origin), gases, and vapours,1 and there is a plethora of guidance on the acceptable limits of common – and not so common – air pollutants that could impact the internal environment. Sources for many of these contaminants may be located indoors – from building components, occupants and occupant activities – outdoors, or both indoors and outdoors.
The range of possible substances is daunting (as comprehensively illustrated in the World Health Organization (WHO) Air Quality Guidelines for Europe2) but, in terms of typical European applications, there are key chemical contaminants3 of carbon monoxide (CO) and nitrogen dioxide (NO2), and the volatile organic compounds (VOCs) formaldehyde, benzene and naphthalene.
There are several of lesser significance, and their impact will be dependent on exposure levels – this, notably, includes carbon dioxide (CO2). Additionally, there are bacteria and viruses, particulate matter (PM2.5, PM1.0 and ultrafine particles) and, in some locations, gases such as radon. Moderating gaseous contaminants and ultrafine particles requires air-cleaning devices in addition to general particle filters.
As explained in CIBSE Technical Memorandum TM40 Health and wellbeing in building services, exposure to air pollutants can have both acute and chronic health effects, from mild to severe – and the pollutants may not even be perceived by occupants. The effects can include temporary discomfort and annoyance (or pleasure) from odours, while some pollutants can have negative impacts on cognitive performance.
Poor indoor air quality (IAQ) can lead to headaches, fatigue, lack of concentration, and irritation of the eyes, nose, throat and lungs; conversely, optimised ventilation providing improved IAQ has been shown to significantly improve cognitive functioning of office workers, including in areas such as crisis response, information usage and strategy.4
TM40 relates to several studies that have found benefits in improving IAQ by avoiding pollutant sources, providing adequate ventilation rates, and filtering the air supply. TM40 refers to ‘a number of studies that found benefits in increasing fresh air rates beyond current best practice recommendations; for example, reducing non-specific symptoms, such as headaches, irritation, or self-reported lethargy, and sick leave’. However, it is noted that most of these studies are inconclusive about the possible causes for the improvements; they do not link ventilation rates with monitored IAQ parameters, and it is therefore not known whether the effects may be related to CO2 levels or to other pollutants.
Part of the cocktail that makes up the IAQ are the variables of air temperature and moisture content, and the consequent relative humidity. These are the comfort parameters that are most readily directly controlled by a mechanical ventilation system, but, in themselves, can trigger events that will impact other aspects of IAQ. For example, high relative humidity will promote microbial growth such as mould, dust mites, fungi, and bacteria, which can have adverse health effects. Higher temperatures and higher humidity levels are linked to increased VOC off-gassing from materials. Maintaining relative humidity between 40% and 60% minimises the risk of microbial growth, as well as maintaining hydrated and intact mucosal barriers of human occupants.
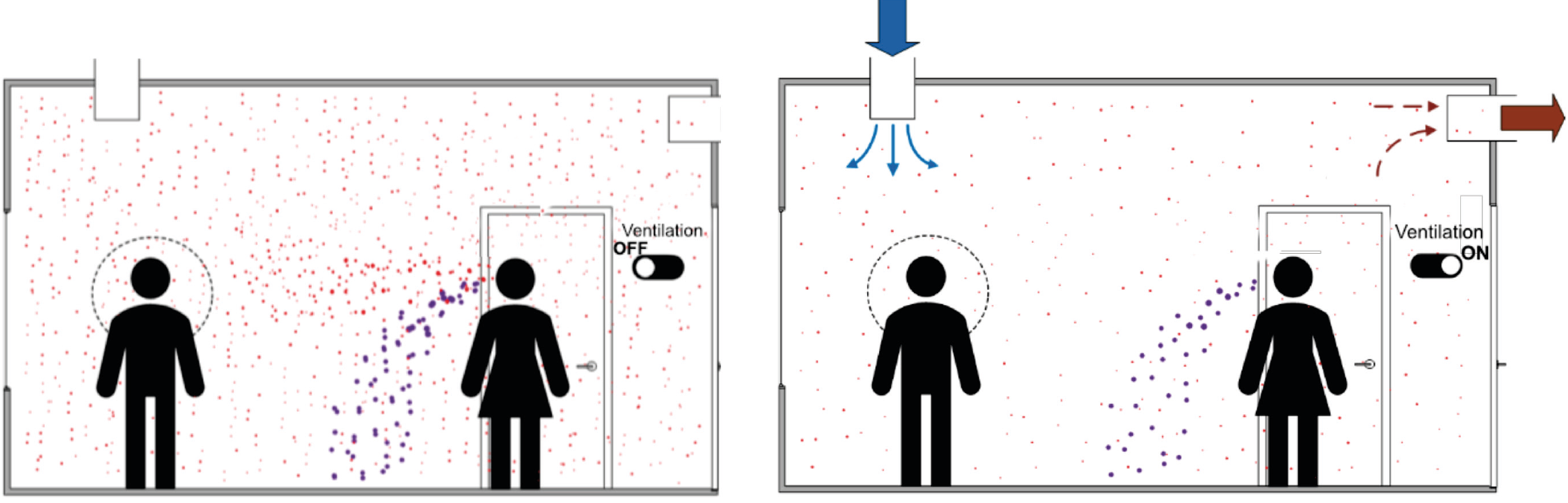
Figure 1: Illustration of how a mechanical ventilation system is likely to impact exposure from an infected person (speaking woman on the right), with aerosol exposure (red dots) in the breathing zone of another person (man on the left). Large droplet exhalation is marked with purple dots (Based on REVHA Covid-19 Guidance6)
The key principles for identifying and controlling potential risks – as proposed in CIBSE TM405 – are ‘source control’ and the ‘precautionary principle’. Ideally, controlling or potentially treating pollutants at source – before they enter the occupied environment – provides the most effective way to reduce risk and avoid reliance on costly, complex, or maintenance-intensive solutions. In terms of precaution, the health effect of design solutions will sometimes only become apparent in the long term, or possibly after they unexpectedly impact a subset of the population.
TM40 emphasises that this does not mean a limit to innovation, but that new methods should be carefully tested prior to application, and a plan should be in place for their monitoring. New solutions should be demonstrated to be safe, rather than waiting for potential evidence that they are not. Any proposed design solutions should be simple to understand and should take account of the expected resources required for operation and maintenance, such as skills, staff and budget.
The predominant advice during the Covid-19 pandemic has been to employ ventilation to dilute and remove contaminants in the indoor air. In many cases, and particularly for domestic and smaller buildings, this may be achievable through increasing the number of open windows and other natural ventilation pathways. However, in larger commercial and institutional buildings, and for buildings located in areas of poor outdoor air quality, mechanical ventilation may present the most practical solution.
Figure 1, based on an illustration in the recently published REHVA Covid-19 Guidance,6 indicates that employing simple mechanical ventilation (with good air mixing) will probably reduce the potential for cross-contamination within a space. When applied with an appropriate ventilation rate, this example illustrates that the risk of cross-infection from SARS-Cov-2 is lowered by virtue of the dilution provided by the incoming (uncontaminated) air – subject to not creating contaminant-laden airstreams connecting the source and receiver.
Therefore, where there is an internal source of pollution – such as SARS-Cov-2 – 100% outdoor-air systems are widely advocated as a means of sustaining low contaminant levels. In most cases, there will be a need for some form of air cleaning to at least protect the air-handling equipment and decorative state of the building, and, increasingly, to reduce outdoor air pollutants that would otherwise adversely affect the health and productivity of occupants.
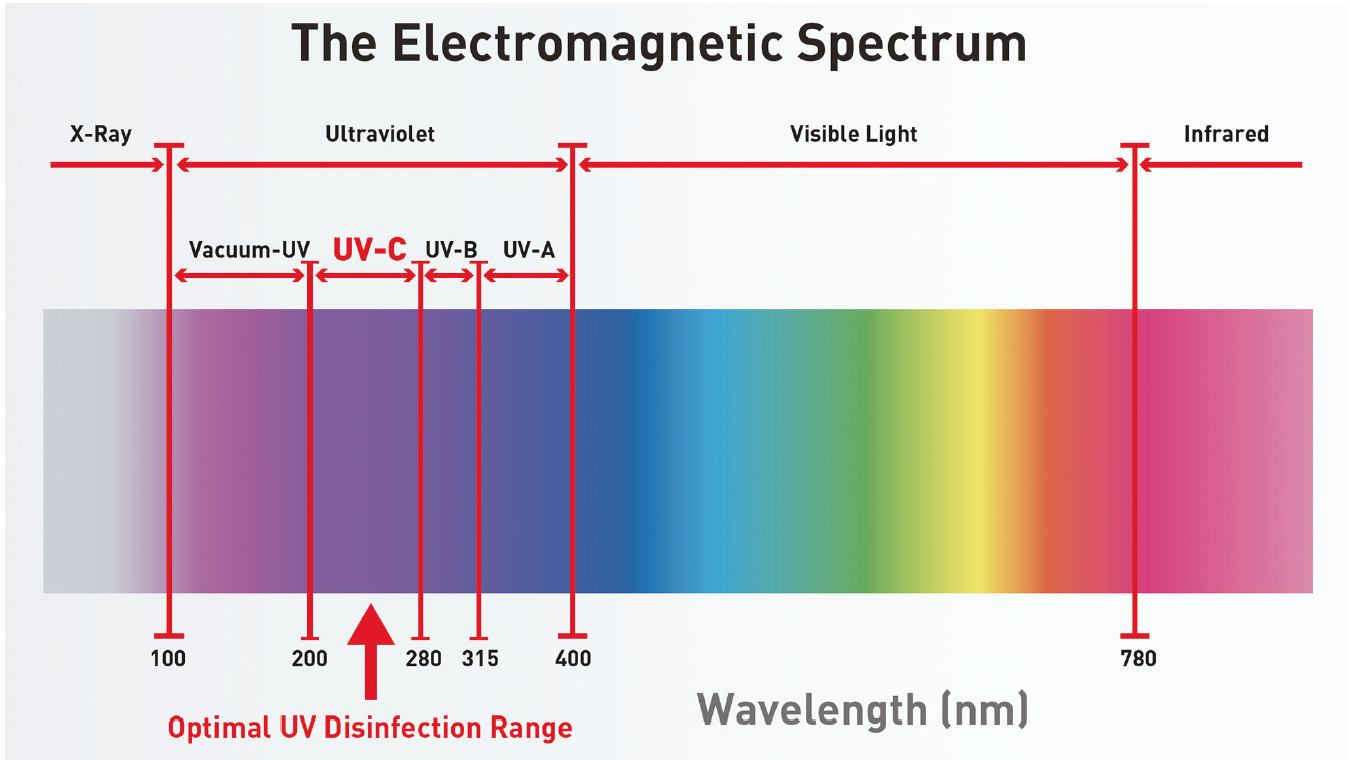
Figure 2: The electromagnetic spectrum highlighting the low-pressure mercury vapour lamp with a main emission at 254nm. Unwanted emission at 185nm generates ozone
Full outdoor-air systems would normally include some form of heat recovery to reduce the heating, cooling and humidification energy consumption. Many systems are designed to employ some proportion of air recirculation (rather than maintain 100% outdoor air), in a compromise between air quality and operational costs. Both full outdoor-air and recirculation systems are designed to supply sufficient air to maintain good air movement in the occupied space, since this will also impact the occupants’ comfort. However, particularly as a result of the pandemic, there is increased concern about the potential risks of cross-contamination between rooms where they are ventilated by shared recirculated air systems.
Research7 published this month (undertaken by the US government’s Pacific Northwest National Laboratory (PNNL) provides an interesting assessment on the impact of ventilation rates, recirculation and filtration on the potential for Covid-19 cross-infection through transmission of aerosols (which can reasonably be extrapolated to other similar airborne contaminants). It confirms that the potential for infection in a single ventilated space reduces significantly as (uncontaminated) air-change rates rise.
When considering multi-space recirculated air systems, the picture is not quite so simple, as higher flowrates from a contaminated room will increase the proportion of contaminants passed into the recirculated air and onto other spaces; the paper provides a detailed, and accessible, explanation of the modelled scenarios. This can lead to a comparatively large increase in the accumulated contaminant levels in the rooms that are supplied with some proportion of this recirculated air.
However, the research determined that, for typical recirculation rates, the introduction of a very basic MERV 8 (≈ ISO Coarse >95%) filter in the recirculated air path significantly reduced the risk of cross-infection, and the modelling indicates that there was little benefit of employing higher-rated filters.
UV-C lamps can be installed at strategic points within the AHU enclosure. In the manufacturer’s example illustrated in Figure 3, the UV-C lamps are employed to decontaminate the coil surface condensate in the drip tray and, within the limitations of the exposure time and UV-C intensity, the air passing through the AHU housing. The lamps are fully enclosed within the AHU’s metal housing, and the units include safety interlocks to avoid accidental exposure to technicians during maintenance.Application of UVGI in an AHU
Many control systems in contemporary heating, ventilation and air conditioning (HVAC) systems are able to provide sophisticated building and zone modulation of ventilation rates. These can typically be programmed to deliver purge ventilation before occupation, so, for example, reducing VOCs that have off-gassed from furnishings and finishes, removing lingering airborne aerosols emitted by occupants during a lunch break, or simply introducing large amounts of outdoor air to stabilise the internal environmental temperatures and humidities to ensure comfortable and healthy conditions immediately before occupation. This can be linked into the control of night-time free-cooling systems that can supplement or replace a purge cycle by precooling the space with outdoor air. In the period after the Covid-19 pandemic, the pattern of working – particularly in offices – may well change, and lead to buildings regularly operating with partial occupancy. Ideally, the HVAC control will allow operation that provides occupancy-based ventilation rates, making more outside air available to occupied zones, thereby ensuring optimal IAQ in occupied areas and reducing energy consumption.
Traditional air-cleaning techniques include different forms of general air filters (classified by BS EN ISO 168908) and EPA, HEPA and ULPA filters (classified by BS EN 18229).
As discussed in the August 2020 CIBSE Journal article,10 the air-pressure drop across a HEPA filter is likely to be two or three times that of a general purpose panel filter. So, existing, and refreshed, technologies have seen accelerated application as building operators seek air ‘cleaning’ devices to moderate the microbial load in the air that do not add significantly to airflow system resistance, system physical dimensions or energy consumption. An example is the integration of ultraviolet germicidal irradiation (UVGI) into AHUs, which inactivates viruses by UV-C photons photochemically interacting with the RNA/DNA molecules in a virus or bacterium, to render these microbes
non-infectious.11
Commentaries on ultraviolet light and its safe application to UVGI are provided in the August 202012 and November 202013 CIBSE Journal.
Modern applications, such as that shown in the indicative panel ‘Application of UVGI in an AHU’ (below), typically employ low-pressure mercury vapour lamps with a main emission at 254nm, which falls close to the peak germicidal wavelengths of 265nm to 270nm. (LEDs are still comparably expensive for such applications.) Some lamps can also emit UV at 185nm (variously categorised as UV-C or ‘vacuum UV’, as shown in Figure 2), which will produce ozone as it splits the oxygen molecules in the air. Ozone is not a desired, or safe, addition to indoor air in occupied spaces, so it is important that such lamps are not used. UV-C will have little impact on moving airstreams where there is a limited ultraviolet dose, as the contaminants swiftly pass by.
UVGI systems do not provide filtration, so inactivated particles, such as dead fungal spores, may remain in the airstream. Therefore, these systems should only be used with a downstream particle filter. Far UV-C light in the range of 200nm to 222nm has been shown14 to disrupt microbes efficiently, but without the potential skin-damaging effects associated with 254nm UV-C. (It can still reach inside viruses and bacteria to cause damage to the RNA/DNA because the microbes are very small.) Far-UVC is likely to evolve into new applications of UVGI.
Whatever the technology, TM40 notes that a monitoring capability should be included in the design, enabling opportunities to gather data on operational indoor environmental quality and on user safety, comfort and satisfaction.
© Tim Dwyer, 2021.
Further reading:
CIBSE Journal CPD Module 27: Indoor air quality and Module 116: CIBSE guidance for delivering good IAQ in the built environment.
CIBSE Knowledge Series: KS17 – Indoor air quality and ventilation
CIBSE TM26: Hygienic maintenance of office ventilation ductwork
CIBSE Covid-19 Guidance: Ventilation (v4)
References:
1 ASHRAE position document on filtration and air cleaning, Reaffirmed by Technology Council 2 February 2021, – accessed 1 May 2021
2 Air quality guidelines for Europe, 2nd Ed, WHO, 2000
3 The INDEX project – Critical appraisal of the setting and implementation of indoor exposure limits in the EU – accessed 1 May 2021
4 Allen, JG et al, Associations of cognitive function scores with carbon dioxide, ventilation, and volatile organic compound exposures in office workers: a controlled exposure study of green and conventional office environments, Environmental Health Perspectives, 1 June 2016
5 TM40: Health and wellbeing in building services, CIBSE 2020
6 REHVA Covid-19 guidance version 4.1, REHVA April 2021
7 Pease, L et al, Investigation of potential aerosol transmission and infectivity of SARS-CoV-2 through central ventilation systems, Building and Environment, June 2021.
8 BS EN ISO 16890-1:2016 Air filters for general ventilation. Technical specifications, requirements and classification system based upon particulate matter efficiency (ePM), BSI 2016
9 BS EN 1822-1:2019 High-efficiency air filters (EPA, HEPA and ULPA). Classification, performance testing, marking, BSI 2019
10 Dwyer, T, Understanding Hepa filters, CIBSE Journal, August 2020
11 www.ies.org/standards/committee-reports/ies-committee-report-cr-2-20-faqs/ – accessed 17 May 2021
12 Pearson, A, UVC lighting – light relief, CIBSE Journal, August 2020 p26-28
13 Young, L, UVGI – Leading light, CIBSE Journal, November 2020 p34-35
14 Buonanno, M et al, Germicidal efficacy and mammalian skin safety of 222-nm UV light, Radiat. Research, April 2017