This CPD will review some of the evidence that predicts the growing need for integrated and efficient heating and cooling for increasingly urbanised living, and will discuss the application of heat pumps employing low global warming potential (GWP) refrigerants as a means of maximising the primary energy resource, and so reducing environmental impact.
Recent analysis1 by Moran et al indicates that 54% of the total global population is clustered in urban areas but accounts for more than 70% of global energy use. By considering carbon footprint data, the research suggests that the top decile of earners drive 30%-45% of emissions, and the 100 highest-emitting urban areas account for 18% of the global carbon footprint.
This will become more challenging as an increasing proportion of the population become urban dwellers in countries with developing economies and in more prosperous countries, such as those in western Europe, where 90% of people are predicted to live in urban environments by 2050.2
The urban heat island effect means that cities are warmer than their surrounding areas. This is primarily caused by meeting the needs of a high population density (comfort and appliances); the structures that absorb and store heat that is re-emitted at night; and limited cooling from evapotranspiration of vegetation, compared with rural areas. The cooling required to maintain comfort and productivity can itself be a significant contributor to the heat island effect, as well as a consequence.
It is estimated3 that air conditioning can raise temperatures by more than 1K overnight in some cities. Higher outdoor temperatures increase the overall need for cooling and reduce cooling system efficiencies (for air-cooled systems), leading to increased primary energy use, higher temperatures and increased use of cooling in a classic feedback loop.4
Across a range of potential urban growth scenarios, Güneralp et al5 estimate that the annual global energy use for heating and cooling may increase 7%-40% from 2010 levels by 2050, so there is plenty of opportunity to seek out improved systems to minimise their environmental impact and cost.
To meet heating and cooling demands, there can be significant benefit in employing heat that is ‘rejected’ from buildings’ cooling systems and processes to meet the thermal loads around the city in an attempt to reduce the consumption of primary energy.
In addition, there is potential for waste heat generated from power stations, the service sector, infrastructure (such as metros and power distribution) and industry, as a by-product of their principal activity. The most recent European Commission report on this, An EU Strategy on Heating and Cooling,6 highlights that, theoretically, enough waste heat is produced in the EU to heat the entire building stock.
In some cases, this otherwise wasted heat can be used directly in the same buildings (or complex), if there are appropriate concurrent heat demands or thermal storage facilities, although, in many buildings, much of the heat cannot be usefully used immediately on site. This presents opportunities to redistribute this heat while, at the same time, being able to integrate large-scale renewable heat sources, using heat networks.
However, the EU’s most recent assessment7 on the uptake of renewable energy sources notes that for heating and cooling ‘the barriers are mainly due to shortcomings related to the capacities of the district heating networks’.
PUE and ERE
The power usage effectiveness (PUE) is defined as the total energy needed for everything used in the bounds of the data centre facility divided by the energy used for computing

Conventional data centres typically have a PUE of about 2.0; many current ‘hyperscale’ facilities have achieved PUEs better than 1.2.9,10
Energy reuse effectiveness (ERE) provides an indication of the useful energy recovery from a data centre that is used, for example, to heat buildings or drive an industrial process (aside that this is used directly in heating and cooling for the data centre itself).

On its own, an ERE of 1.0 does not imply an efficient data centre infrastructure – PUE and ERE together provide a better impression of the performance of data centres that reuse energy.
See ERE: A metric for measuring the benefit of reuse energy from a data center11 for a more detailed explanation of the evaluation of ERE.
Currently, district heating provides 9% of the EU’s heating; in 2012, the main fuel was gas (40%), followed by coal (29%) and biomass (16%).6 District heating can integrate renewable electricity (through heat pumps) by exploiting natural heat resources, such as geothermal, ground and water sources.
Also, as stated in the European Commission document,6 ‘synergies between waste-to-energy processes and district heating/cooling could provide a secure, renewable and, in some cases, more affordable energy in displacing fossil fuels.’
Driven by F-Gas legislation for new low-GWP refrigerants – and the requirements of Ecodesign and standards such as BS-EN 148258 to meet increasingly robust efficiency requirements (as measured by seasonal coefficient of performance (SCOP) and seasonal energy efficiency ratio (SEER)) – heat pumps have been designed that are capable of delivering relatively high hot-water temperatures at high system efficiencies.
This has increased the opportunity to employ such systems so that large heat producers – for example, data centres – can be used to provide heat for heat pump-powered district heating systems. The prime objective in controlling a data centre environment is to provide the correct amount of cooling, as efficiently and as reliably as possible, so as to allow the computing equipment to operate most effectively.
The operational effectiveness, in terms of energy, is typically measured in terms of its power usage effectiveness (PUE), although there is increasing interest in the additional evaluation of energy reuse effectiveness (ERE), which indicates how otherwise wasted energy is used (see boxout ‘PUE and ERE’).
Traditionally, cooling has been provided using mechanical vapour compression cooling, with the heat being rejected to the outside atmosphere through an air, or water, heat-rejection system. As data centre cooling represents a significant proportion of the building’s energy consumption, and is typically considered to be around 40% of total energy use, there is an increasing focus on improving the efficiency of cooling solutions to reduce the real PUE and provide a smaller ERE.
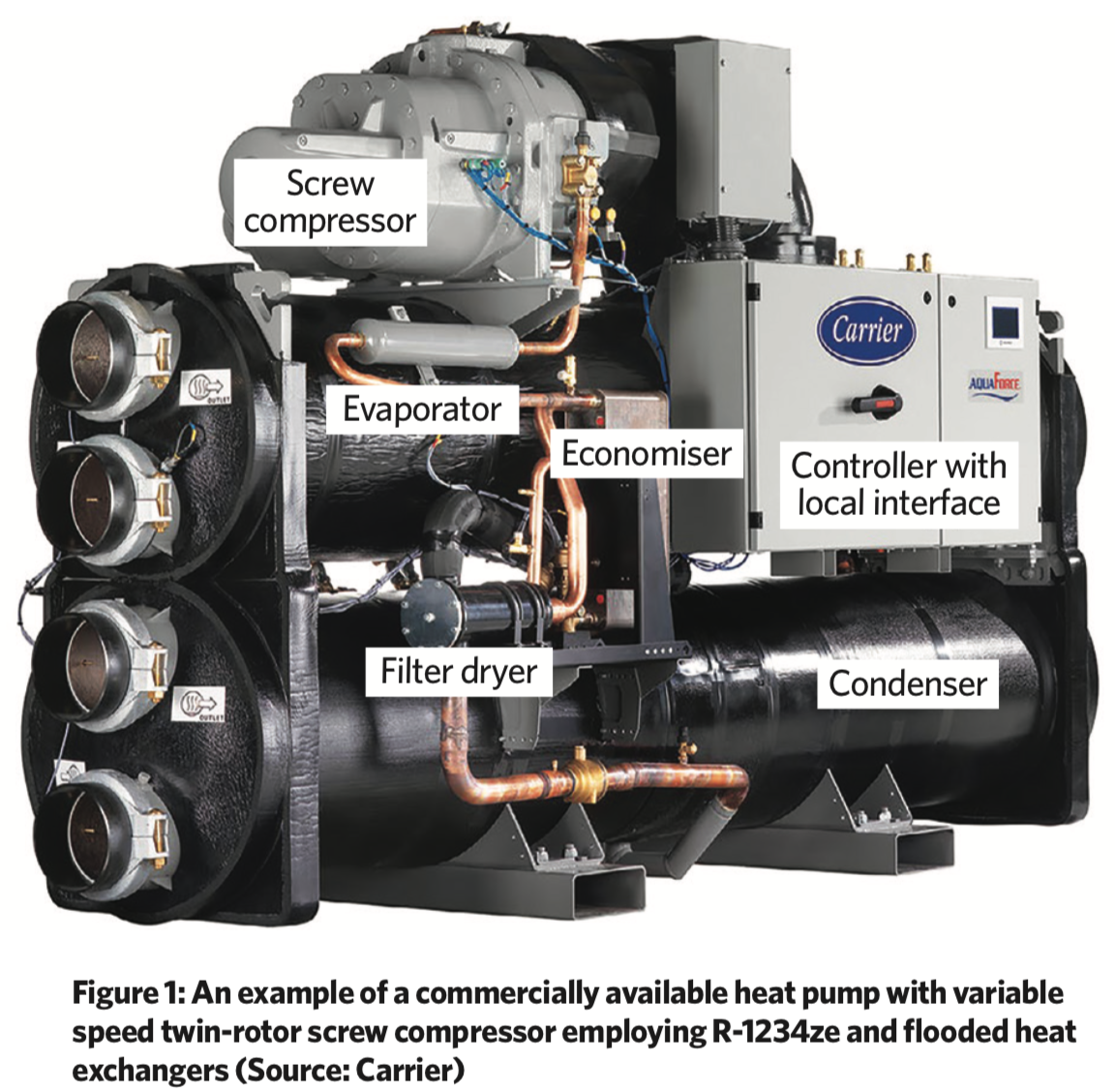
Whether using mechanical cooling equipment alone or in combination with evaporative or air-based cooling systems, this still involves ‘rejecting’ heat to the local environment. A common design approach is to recover the heat directly from the cooling system at the relatively low temperatures associated with vapour compression refrigeration, using heat recovery condensers or desuperheaters added between the compressor and the condenser.
Using heat-recovery condensers, such systems can achieve water temperatures up to 50°C or, when using desuperheaters, up to 60°C (but with significantly less heat available in the latter case). Depending upon the application, such water temperatures, combined with the significant amounts of available heat, can offer economic advantages and reduce the ERE.
However, as the rejected heat is typically not at temperatures required for many district heat networks, heat pumps can be applied to deliver the heat at a more usable temperature. Modern refrigeration technology and low-GWP refrigerants such as the hydrofluoroolefins (HFOs) can provide heat pumps capable of delivering hot water up to 85°C.
The temperature that a heat pump, such as that shown in Figure 1, is able to produce economically will be dependent on the temperature of the source heat, as well as the design and operational characteristics of the heat pump.
Manufacturer’s data12 indicates that, for example, the heat pump in Figure 1 can economically deliver temperatures of 85°C (COP 2.26) at a modest source water temperature of 20°C (leaving the evaporator at 15°C). The COP of the heat pump system can be significantly improved by employing two modular heat pumps in series, as illustrated in Figure 2.
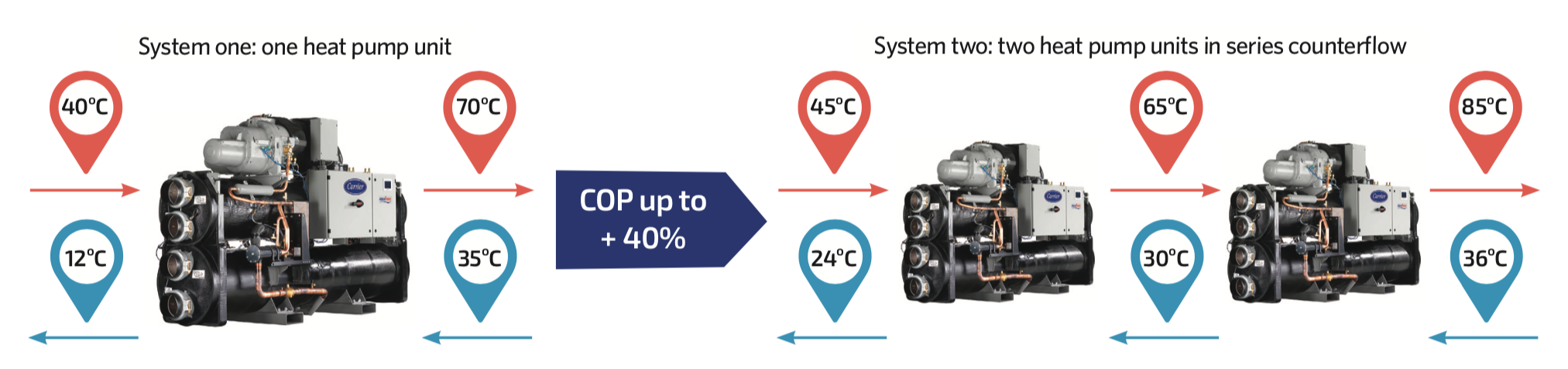
Figure 2: A comparative example of the impact on the COP of using single and dual-series connected screw compressor, flooded heat exchanger, HFO heat pumps (Source: Carrier)
Series-connected heat pumps can economically deliver temperatures that are associated with standard low-temperature hot water heating from a relatively low temperature source. These can provide an efficient means of heat recovery, either as stand-alone installations or to supplement traditional boilers in applications such as district heating or industrial processes.
By using ultra-low GWP refrigerants, such as HFO R-1234ze, in well-designed and installed systems, the potential environmental impact is reduced significantly compared with previous-generation hydrofluorocarbon (HFC) refrigerants.
Such systems can simultaneously produce chilled and hot water to supplement boilers as well as replace chillers, and attain an operating COP of 3.0 or more, so benefiting the ERE.
In the emerging smart cities, there are opportunities to pool the thermal resources of the disparate users so that the net heat producers reduce primary energy use in other parts of the city that require heat. As illustrated in Figure 3, buildings that have excess heat connect into the heat recovery (cooling) loop that broadly maintains the temperature (and so the exergy) of the ‘waste’ heat.
This loop supplies the evaporator of the separately located heat pumps that can supply water (from the condenser) at, for example, 85°C to the district heating loop.
The low temperature ‘heat recovery’ flow and return can extend to a number of nodes, where discrete, individual heat pump substations can supply heat at the required temperature to local groups of loads.
There are several well-established projects in Europe that take the heat from data centres in this way to meet residential and commercial heating loads, which have displaced the need for numerous individual fossil-fuelled boilers.
The end users benefit from a more sustainable, metered, reliable supply of heat without the need to host and maintain heating plant – and, by spreading the loads across districts and cities, the variabilities of overall load demand are reduced and efficiencies maintained.
The same concept may be usefully applied where there is sufficient diversity of load in a single building (or development), to effectively maintain its own self-contained heat networks.
As described by Nick Boid13 in the June 2016 CIBSE Journal, an example of this is the Riverlight development, adjacent to the River Thames near Battersea, London, which includes 812 residential apartments, retail sites, restaurants, bars, leisure facilities and a child daycare centre. To balance the load requirements, this scheme employs an open loop ground source of eight deepwater wells to store and recover energy that is linked, through plate heat exchangers, to the heating and cooling systems.
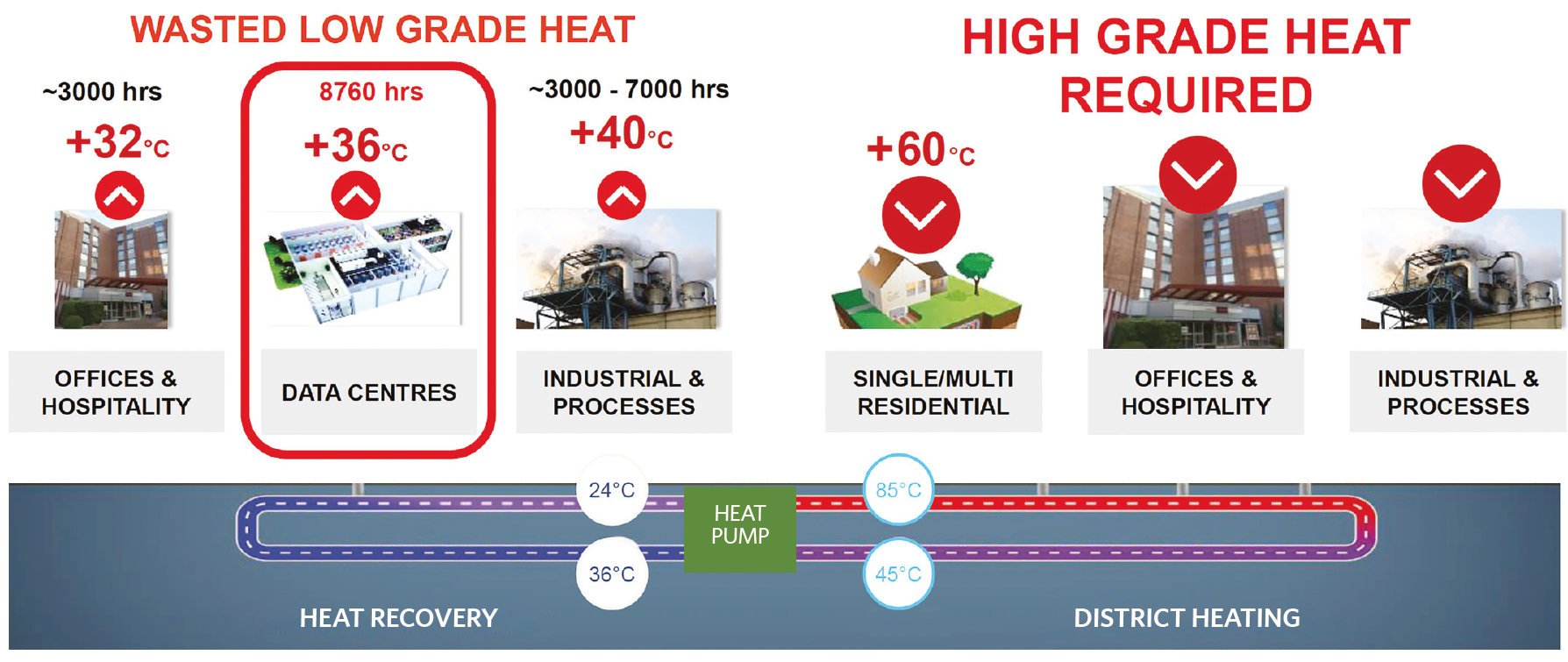
Figure 3: City or district heat recovery loop boosted with heat pump to provide district heating, showing typical annual hours of heat availability (Source: Carrier)
There are three 500kW R134a water-to-water heat machines that are controlled to provide optimum conditions to produce hot water or chilled water to meet the overall loads. A 60m3 central thermal store integrates the heat generated from the ground source heat pump and the site’s combined heat and power (CHP) plant to serve the distributed heating systems. In heating mode, the heat pump produces hot water up to 45°C, and can operate with a COP of more than 6.5 because of the availability of the warm water ground source.
In cooling mode, the system produces chilled water for comfort cooling, using ground water as a condensing medium. When a chilled water supply temperature of around 10°C is required, direct cooling from the wells may be used and, alongside some heat pump power, deliver a combined energy efficiency ratio (EER) of 10. In mixed mode, the system provides cooling for the building, while also catering for the domestic hot water requirements.
The successful integration of heat pumps into city- or district-wide systems undoubtedly requires a holistic design approach, to ensure that the application is appropriate and that all affected parties are involved, so it meets their needs while delivering high levels of operational effectiveness, low environmental impact and good value.
However, if planned and executed correctly, there are potentially many opportunities where the smart application of heat pumps can deliver high-performance district heating and cooling schemes, while reducing primary energy use.
© Tim Dwyer, 2019.
References:
1 Moran, D et al, Carbon footprints of 13,000 cities, Environmental Research Letters, Volume 13, Number 6, 2018.
2 2018 Revision of World Urbanization Prospects, United Nations, 2018.
3 Salamanca, F et al, Anthropogenic heating of the urban environment due to air conditioning, Journal of Geophysical Research, Vol. 119, Iss. 10, 2014.
4 The Future of Cooling – Opportunities for energy-efficient air conditioning, OECD/IEA 2018.
5 Güneralp, B et al, Global scenarios of urban density and its impacts on building energy use through 2050, PNAS, 2017.
6 An EU Strategy on Heating and Cooling, EC, 2016.
7 EC COM (2019) 225 Renewable Energy Progress Report, EC, April 2019.
8 BS-EN 14825:2018 Air conditioners, liquid chilling packages and heat pumps, with electrically driven compressors, for space heating and cooling – Testing and rating at part-load conditions and calculation of seasonal performance, BSI 2018.
9 Jones, N, How to stop data centres from gobbling up the world’s electricity, Nature, 12 September 2018 – accessed 23 July 2019.
10 Google Data Centres: Efficiency: How we do it – accessed 9 August 2019.
11 ERE: A metric for measuring the benefit of reuse energy from a data center, The Green Grid 2010.
12 Performance Report (full-load) Water to Water Heat Pump, 61XWH-ZE14, Carrier, August 2019.
13 Boid, N, Lightening the load, CIBSE Journal, June 2016.