This CPD will consider some of the evidence that has confirmed overheating as a significant problem, and will introduce CIBSE’s TM59, which provides a common set of parameters to predict overheating risks realistically and establish when the risks of a particular design would require the inclusion of active cooling.
As highlighted1 by the UK’s Committee on Climate Change, there are currently around 2,000 heat-related deaths in the UK each year, with much of the increased risk thought to be caused by exposure to high indoor temperatures. BRE’s guidance2 reported that the 2003 heatwave in Europe resulted in more than 20,000 heat-related deaths, with 2,000 in England alone. In addition, there are predictions of significant increases in UK heat-related mortality3, rising from 2,000 deaths per year in 2015 to an estimated 7,000 per year by the 2050s. Mortality is an extreme consequence of overheating, but poor thermal comfort, illness, stress, anxiety, sleep deprivation and reduced productivity and wellbeing can all stem from excessive internal temperatures.
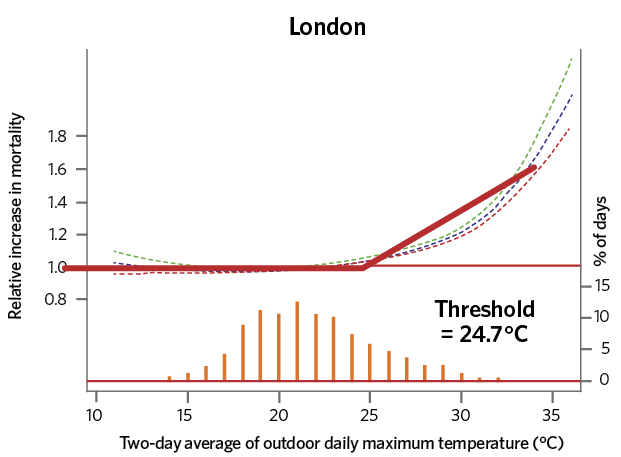
Figure 1: Relative increase in mortality for London inhabitants related to temperature, together with the banded two-day London historic external maximum air temperature5
In a study of the risk of heat impacts for London, Katie Jenkins4 suggests that, by the 2030s, between 59% and 76% of flat-based residents – and 24% to 29% of people in detached properties – could experience overheating during a heatwave. For the 2050s, under assumed projections of change in climate and land use, the values potentially increase to between 80% and 92% in flats, and 56% to 61% in detached dwellings. The estimates of cost rise from a total annual figure of £10m to £50m today, to between £25m and £150m per year by 2050 – and £40m to £350m by 2080.
As highlighted in the recently published CIBSE Technical Memorandum 59 Design methodology for the assessment of overheating risk in homes, overheating is dependent on multiple coincident factors. Whether a person suffers heat stress will be influenced by a whole gamut of parameters that relate not only to the actual temperature of their environment, but also to the many other influences that impact on human thermal comfort. This means that not all occupants will concur with their individual environmental assessments, but it indicates the need for a benchmark methodology to undertake meaningful comparisons and form reliable design decisions.
As cited in the literature review5 for the UK government’s assessment of the risks of overheating in homes, work undertaken by Armstrong shows that the impact (on mortality) of high temperature varied in the different regional climates of the UK. The London area was shown to have the highest threshold and, coincidentally, the highest mean summer temperature (which might indicate a degree of personal adaptation). London data shown in Figure 1 indicates an upturn in mortality beyond an external temperature of 24.7°C. Armstrong’s work noted that, when attempting to model the impacts, the prediction was improved when it accounted not only for the daily maximum temperature, but also for the daily minimum temperature.
Reducing the opportunity for overheating
Around 45% of buildings professionals estimate2 that there is ‘little or no additional cost’ to incorporating passive cooling measures in new buildings at the design stage. Design measures2 for homes include: avoiding highly glazed south-facing façades; ensuring a good ventilation-to-floor-space ratio; external shutters; trickle vents; green roofs; and green walls covered in vegetation. Use of passive measures should be the primary means of reducing the risks of overheating and, in many cases, these will be sufficient.
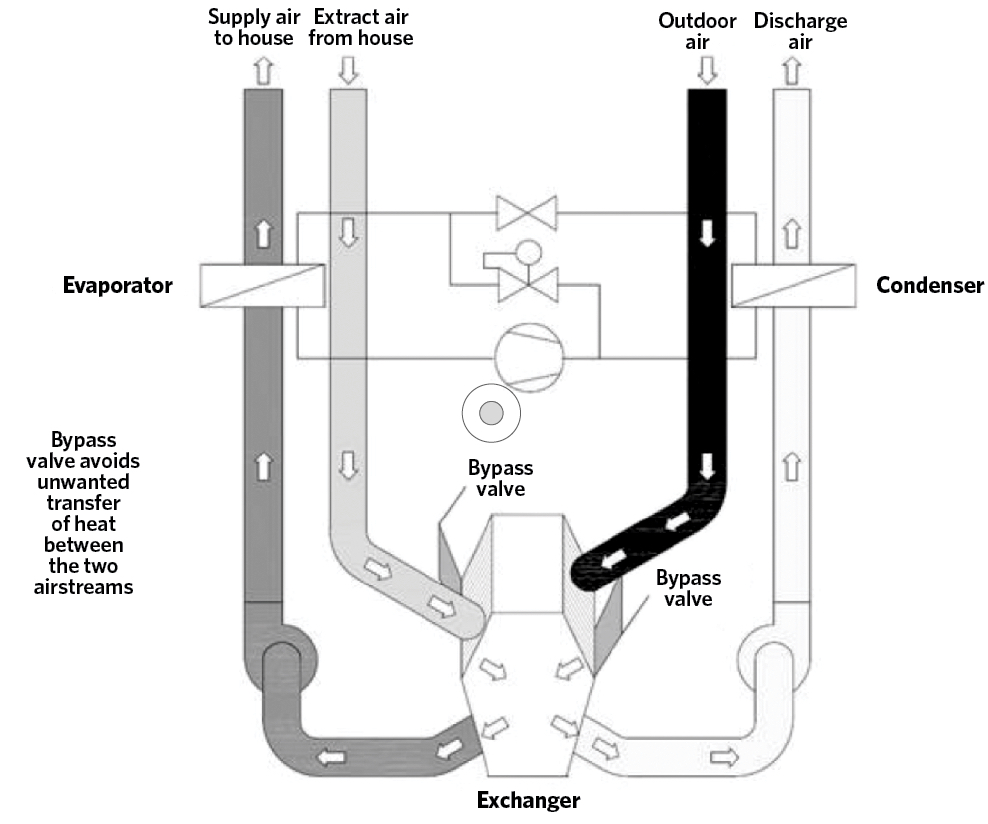
Figure 2: Home mechanical ventilation and heat recovery system integrated with a ventilation system heat pump (Source: Zehnder)
A properly designed and operated mechanical, whole-house ventilation system that supplies and extracts air can ensure air movement around the property, as well as significantly enhance the ventilation rate. When outdoor air is cooler than indoor air, a cooling effect can be realised. Through the integration of a heat exchanger with bypass – to form a mechanical ventilation with heat recovery (MVHR) unit – the supply air temperature may be moderated throughout the year.
There are likely to be residential applications that require some form of augmented cooling to maintain reasonable conditions. A number of possibilities can be explored for further cooling the air, some of which are likely to be more suited – and cost-/energy-effective – when applied in systems serving multiple homes. The following are examples of systems that can also be used in conjunction with a heat recovery device:
Ground pipes – where the outdoor air is drawn through underground cavities or pipes.
Ground to air subsoil exchanger – where the outdoor intake air is brought through a heat exchanger containing a brine solution that has circulated around a ground coil.
Indirect evaporative cooling – when the incoming air is passed across a heat exchanger where, on the other side, external air has been evaporatively cooled (and that cooling humid air is then directly discharged to the atmosphere).
Surface water cooling – where the outdoor air exchanges heat with water drawn from a water mass, such as a lake, river or the sea.
Evaporator coil in the airstream – containing a cold, low-pressure refrigerant that rejects heat to the external environment via a heat pump/refrigeration process.
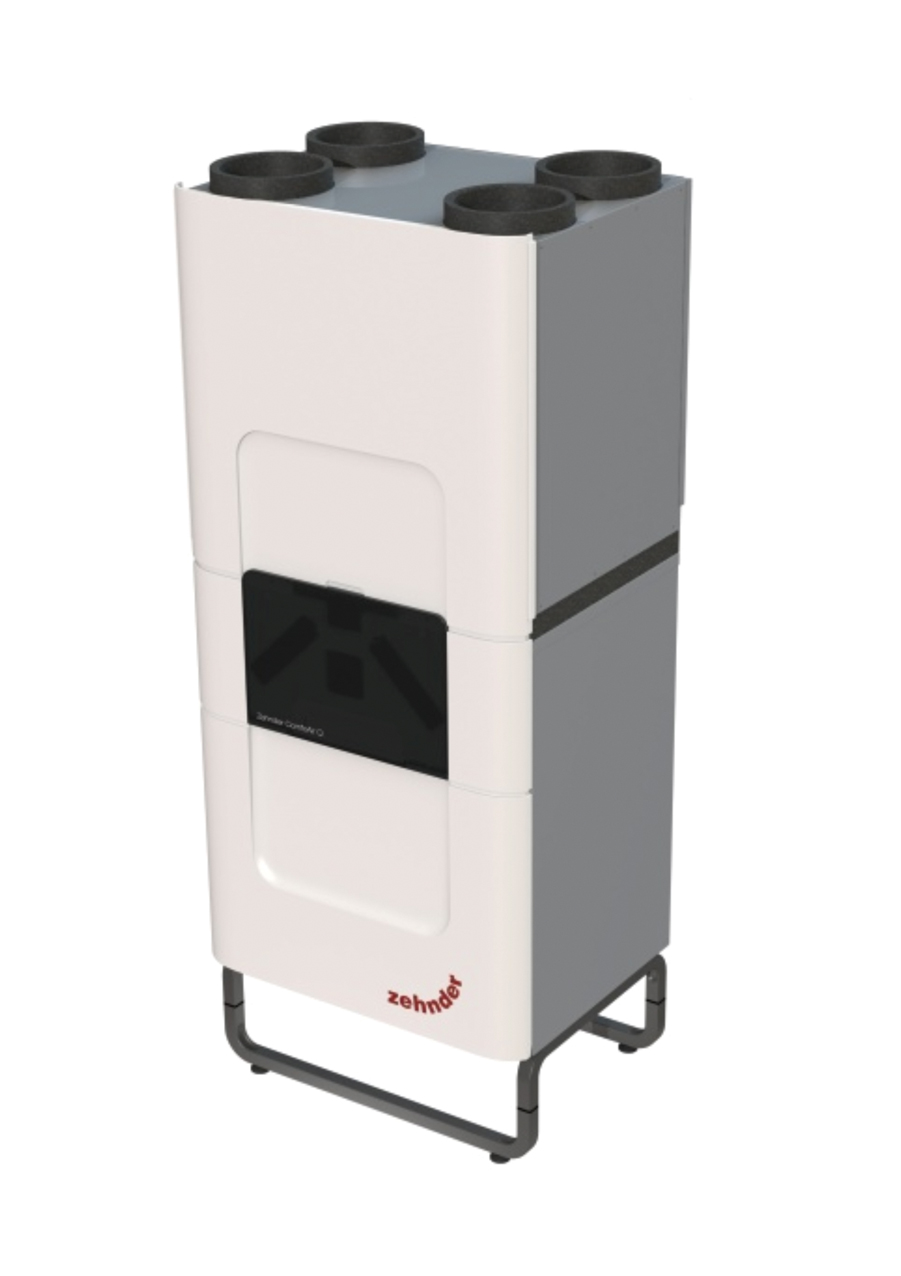
Figure 3: A stand-alone unit that supplies cooling to the incoming air and encloses all the required vapour compression refrigeration components in internally mounted housing, connecting with a MVHR system. Heat is rejected to the discharge airstream. (Source: Zehnder)
As part of a US study6, Jennifer Bobb considered the impact of air conditioning as a factor in the incidences of heat-related mortality in US homes. The analysis is not clear-cut, as there are other factors that could have affected the results – such as high air conditioning operating costs on warmer days discouraging use of the systems; and not knowing whether the more vulnerable in the population were those who had actually installed air conditioning. However, the findings indicate a relationship between those US regions with increasing applications of home air conditioning and reduced mortality, including the more temperate areas of the north-western and north-eastern US, which have similar climates to the UK. This indicates that air conditioning (or augmented cooling) could be used in applications where passive measures and outdoor ventilation alone are insufficient to reduce overheating risk adequately. This will incur operating costs, increased energy use and increased urban heating.
Chapter 5 of the recent UK Climate Change Risk Assessment 2017: Evidence Report cites accounts indicating that the application of dwelling air conditioning is currently low in the UK, at 3% of homes. This is likely to increase significantly, although, it notes, this would require increases in system energy efficiency and reductions in the installed costs compared with standard air conditioning systems.
Where other methods – including passive measures and MVHR – are impractical or simply unable to maintain reasonable conditions, compact systems, such as that schematically illustrated in Figure 2, are being marketed as a means of providing active cooling, as well as ventilation, to meet this challenge. They employ packaged vapour compression refrigeration systems that cool the supply air when needed and benefit from unitary construction that integrates into whole-house ventilation systems.
Determining the risk
CIBSE’s TM59 domestic overheating risk assessment methodology has been developed – and tested on typical UK projects – to facilitate robust modelling of overheating risks for homes that, although based on UK data, could be evolved for application in other temperate climate zones. It provides a standardised set of inputs and associated methodology that can be applied with commonly used dynamic thermal modelling tools. TM59 is not, in itself, a calculation method, but is guidance to encourage good design that delivers comfortable environments within sensible limits, without being so stringent that it over-promotes the use of mechanical cooling.
The method prescribes standardised inputs that include occupancy profiles, internal gains, window opening and shading profiles, and external design conditions (based on the CIBSE TM59 Design Summer Year, DSY01, 2020s High-emissions 50% scenario) so that meaningful comparisons may be undertaken, based on a common set of conditions. Infiltration and mechanical ventilation rates are based on the particular design parameters for the home under consideration, including natural ventilation if feasible. Residences that are predominantly mechanically ventilated must satisfy the comfort criteria without the MVHR units being in ‘boost’ mode, which can cause noise issues. An unwanted side-effect of this requirement could be to increase the ventilation rates for normal (unboosted) MVHR systems in new homes (operating 24/7), which may have detrimental effects on the building energy use.
Profiles for the occupancy and equipment gains were developed specifically for TM59 to account for when overheating risk is at its highest – in the early afternoon (likely peak gains) and during night-time (when sleep may be disrupted) – based on occupancy for 24 hours a day, seven days a week, May to September. The profiles provide a common basis for comparison across all assessments that will all include:
- Occupancy – derived from CIBSE and ASHRAE data. Bedrooms are assumed to have 24-hour occupancy and other spaces daytime (13 hours per day) use only.
- Solar gains through glazing – any assumed shading must be confirmed as being actually fitted and in use.
- Equipment – the common appliance profiles (in watts) are based on the number of occupants in the residence.
- Lighting – proportional to floor area, but accounting for automatic control. Night-time (6pm to 11pm) is assumed as 2W·m-2.
Larger or unusual apartments follow the same principles and can apply alternative profiles for specific room types, but these should be clearly identified in the resulting compliance report. The TM59 data and method can also be a useful starting point for the assessment of more extensive institutional accommodation, such as care homes and prisons.
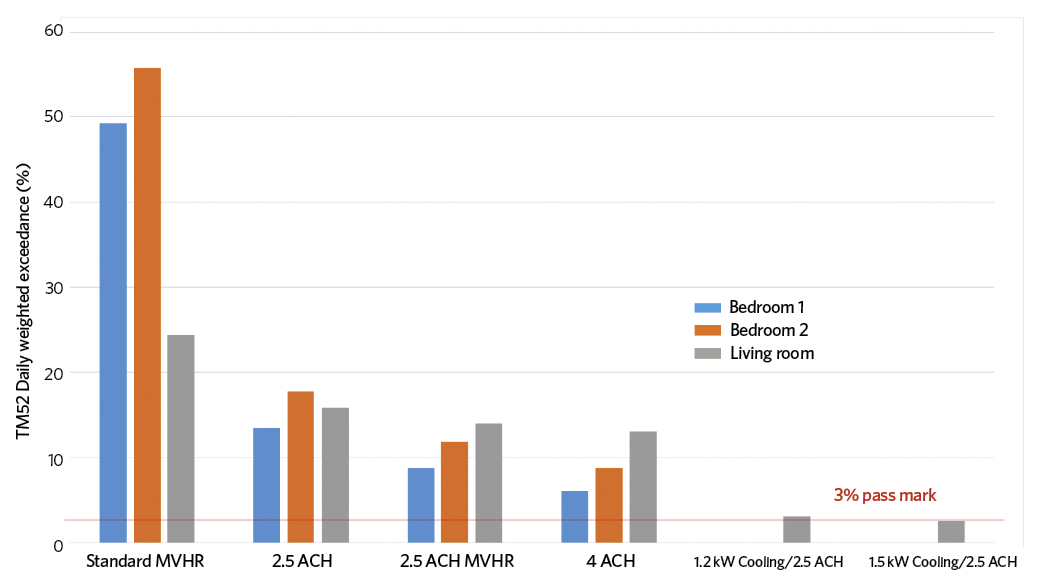
Figure 4: The results of modelling a two-bed, mid-floor apartment using parameters derived from TM59 to explore overheating risk (Source: Zehnder)
To satisfy the recommendations of TM59 for naturally ventilated homes, modelled room operative (comfort) temperatures should not exceed the upper comfort limit by 1K for more than 3% of summer occupied hours. Bedrooms should not exceed 26°C – the temperature above which sleep patterns are disturbed – for more than 1% of the annual hours between 10pm and 7am. For mechanically ventilated homes, the operative temperature should be no more than 26°C for 3% of annual occupied hours. It is not mandatory to comply with TM59; however, the overheating risk should be reported as part of the overall building performance assessment.
When assessing projects with multiple housing units, the highest-risk dwellings should be identified and assessed. Typically, these include single-aspect, highly glazed, top-floor units with limited window openings. Two case studies of applying TM59 methodology are included in Ashley Bateson’s July 2017 presentation, and show the efficacy of passive design measures and MVHR in controlling overheating risks for those specific examples (an apartment block adjacent to a busy road and a set of exposed high-rise apartments).
The output from a further TM59 modelling example is shown in Figure 3. This is for a 73m2, two-bed, mid-floor apartment with a single 39m2 south-facing – and a 4.5m2 west-facing – external brick façade
(U = 0.18W·m-2K-1) with a total of 13.2m2 glazing (U = 1.4W·m-2K-1, g = 0.6, 15% frame) with no external shading. The apartment includes a combined kitchen and living space, and a bathroom, as well as the two bedrooms, and has a design airtightness 4m3·h-1 @50Pa per square metre of build envelope.
The apartment model was produced for a project located in southern England, and the CIBSE 2016 Southampton DSY1 2020 50th percentile high-emissions scenario weather data was applied. The analysis indicates that, for this particular, challenging application, a standard or oversized MVHR is unable to meet the required overheating criteria that cannot be met by passive means alone. By applying 1.5kW of cooling – for example, with a compact heat pump cooling unit, as in Figure 3 (with the condenser rejecting heat into the discharge air, as in Figure 2) – the apartment is likely to meet the requirements of TM59, with an air flowrate of 2.5 air changes per hour.
© Tim Dwyer, 2018.