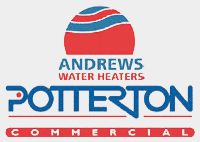
The conjunction of changes in Part L of the Building Regulations, impending European legislation prompted by the EuP (Eco-Design of Energy Using Products) Directive and the Code for Sustainable Homes are placing ever increasing demands on improved appliance and system efficiency for space heating and hot water generation.
Building heating and hot water systems are more frequently becoming ‘bivalent’ with a combination of heat sources being deployed in plant rooms to deliver the carbon savings demanded by local authorities and the underpinning legislation. Commercial heating and hot water systems will inevitably need to include a combination of conventional products such as boilers, directfired water heaters and calorifiers married with more innovative technologies such as solar thermal, heat pumps and combined heat and power (CHP) in order to deliver low carbon plant room solutions.
The UK government’s Renewable Heat Incentive (RHI) is planned for introduction in April 2011 and is aimed at supporting heating at all scales, including households, businesses, offices, public sector buildings and industrial processes.
The ‘clean energy cashback’ tariff levels proposed by the RHI for biomass, biogas, heat pumps and solar thermal vary according to installed system size. Ground source heat pumps up to 45 kW will qualify for tariffs of 7 pence/kWh; between 45 kW and 350 kW, 5.5 pence/kWh and those above 350kW will qualify for a tariff of 1.5 pence/kWh. The two lower bands would not require any metering of energy supplied to the building by the GSHP (being based on installation size and deemed annual generation) and the payments would be made for at least 20 years. Despite the proposed legislation being a year away systems that are installed now (that are certified and registered under the Microgeneration Certification Scheme, MCS) would benefit. The MCS (or equivalent schemes accredited under EN 45011), certify microgeneration products and installers in an attempt to provide consistent European standards.
The principle for ground source heat extraction
The ground source heat pump is driven by solar radiation that, in this case heats the ground; as well as the continuous flow of heat from the earth’s core. As a result, the ground temperature shows seasonal fluctuations to depths of about 15 m where the temperature is approximately equal to the mean annual air temperature (8 – 11°C in the UK).
Figure 1: Approximate UK Annual Ground Temperatures
The temperatures above this will alter throughout the year as illustrated by the graphs in Figure 1. Ground source heat pumps use this stored energy to provide a thermal source for one side of a refrigeration cycle recovered through the use of a ‘ground loop’ that draws its heat from either ‘open’ or ‘closed’ ground loops. This would most frequently employ a vapour compression refrigeration cycle. The available heat generated (and released from the condenser of the refrigeration cycle) is then used for low temperature space heating or supporting the generation of hot water.
There are two heat exchangers in a GSHP: Source side – the evaporator: A ground loop manifold provides the interface between the fluid that is drawing the heat from the ground and the source side exchanger as shown in the example in Figure 2. Load side – the condenser: Transfers energy between the high pressure, high temperature refrigerant gas (post compression) and the heating/hot water circuit.
The complete heat exchange cycle is shown in Figure 3.
Figure 3: The heat exchange process in a GSHP system
The heat transfer fluid is a water/glycol mix to prevent freezing during periods of low ambient air temperatures and ground frost.
Open and closed loops
Water that naturally flows through the ground in aquifers (in spaces between gravel, sandstone, and fractured rock) will tend towards the temperature of the ground through which it is flowing. By drilling holes into the aquifer, the depth of which will vary depending on location, the water can be diverted (pumped) to provide a source of heat for the heat pump evaporator. This is known as an open loop system and to determine the availability of such a resource requires a specialist hydrogeological survey to determine the extent and longevity of the available resource.
As a consequence of the more complicated survey requirements of this technique and due to greater uncertainties (such as unforeseen geological conditions, air locking and collapse of the boreholes or a falling water table), closed loop systems are more frequently employed where the heat exchanger is constructed within the ground itself. Closed loop heat exchangers usually consist of a sealed loop of polyethylene pipe containing a heat transfer fluid which is pumped around the loop.
The heat exchanger may be installed vertically or horizontally, the choice of the arrangement will depend on the available land, local soil type and excavation costs. Like open loops they can still suffer from air locks and they require careful protection both during installation and after they have been installed to prevent blockages.
Closed loop ground heat extraction using a horizontal trench
Where horizontal trenches are used to extract energy from the ground, then the depth would normally be between 1.5 and 2 metres. For strip trenches (used commonly with ‘slinky’ type coils) the width of the trench is typically the same as that of a bucket on a digger.
The actual area required for the ground loop will depend on the type of ground and the annual heat extraction period. Based on Central European data EN 15450 provides the guidance in Table 1.
So, for example if a load of 10kW was to be drawn from ground that is made up of wet clay for around 2,000 hours per year it might be reasonable to use a value of 25 W/m2. Hence the ground loop should cover a ground area of 10,000/25 = 400m2.
The pipe should be laid no closer than 0.8m apart and so this would mean a pipe length of at least 400/0.8 = 500m. To ensure that there is not excessive pressure drop in the ground loop (to both reduce operating costs and maintain reasonable pressures) the practical pipe length is related to the pipe diameter. The recommended maximum length for 25mm is 100 metres and for 32mm pipe is 200metres so in this case if 25mm pipe was used then four loops would be used linked up via a manifold (as in Figure 2).
Figure 2: Manifold for connecting multiple ground loops to evaporator heat exchanger
Closed loop heat extraction via deep bore hole
Vertical bore hole based ground loops are suited to applications where there is limited ground availability as in more densely populated areas but are more expensive to install. Bore holes are required to be deep (in the order of 50 to 100 metres) to accommodate the appropriate length (and hence surface area) of pipe to extract the required heat from the ground. The bore holes would normally be no closer than 6 metres apart and the pipe would be surrounded by a grouting medium (injected at the same time as the pipe is inserted) to ensure good heat transfer between the ground and the pipe. The amount of heat that can be extracted from the borehole is determined by the type of ground and the annual operation period. Table 2 provides an abstract of the data derived from applications in Central Europe giving the approximate available heat that may be extracted per metre of vertical borehole.
And so using the same 10kW load as before the extraction rate would be just under 60W/m and so the vertical borehole would need to be 10,000/60 = 167 metres deep. Practically, two bore holes of approximately 85m deep or three at 60 metres are likely to be used. In recent years there have been a growing number of ground source loops being integrated into the piles of buildings.
Whether the ground loop is open or comprises horizontal trenches or deep bore holes, it is always recommended to conduct a geological survey.
Optimising operational performance
The key aspects to ground source heat pumps delivering economic and environmental benefits is control of its operation. The Coefficient of Performance (COP) of the heat pump is a function of the ground loop temperature and the load into which the thermal output of the heat pump is being discharged – the closer the two temperatures are the greater the COP and COP may be calculated from:
COP = thermal heat delivered (kW) / electrical power supplied (kW).
As the temperature of the ground loop is relatively constant, unlike air source heat pumps, seasonal variations in performance with a ground source heat pump will be less than for air source. For comparison purposes the performance of heat pumps is frequently measured on a seasonal basis using the seasonal performance factor (SPF) and
SPF = heat energy delivered (kWh/ season) / electrical energy supplied (kWh/season).
Figure 4: ‘Slinky’ coil being installed
As electricity is being consumed and thermal energy is being generated, the financial benefit offered by ground source heat pumps is a function of the electricity tariff and the cost of the fuel being used as a primary source of heat (gas, LPG, oil or electricity). In a properly installed GSHP the heating energy costs should be below that of any other centrally supplied fuel.
The better COP is achieved in applications where the ground source heat pump is integrated into a heating system using low temperatures such as under-floor heating, such as the one shown in Figure 5.
Figure 5: Space heating (under-floor) application
Another application to ensure low flow and return water temperatures to the heat pump condenser is to use the heat pump to pre-heat hws feed water where, using an indirect cylinder as an interface, the ground source heat pump can operate in conjunction with a direct-fired water heater or a hot water calorifier as shown in Figure 6.
The schematic shows the ground source heat pump load side temperatures being controlled to 35°C flow and 25°C return. At these operating conditions temperatures within the pre-heat cylinder can rise to 25°C. This reduces the amount of fuel required in the primary heating appliance to raise the hot water service to 60°C with a subsequent reduction in energy costs and carbon emissions.
Additionally the use of a the indirect heater will provide some buffer storage – this is beneficial as it is not recommended that ground source heat pumps start up more than three times in a one hour period. If a preheat cylinder is employed then particular care must be taken when determining operational requirements to avoid legionella.
In under-floor heating applications, if the size of the heating array is relatively large, holding a high volume of water, this may act as buffer storage and could mitigate the need for the buffer vessel. This needs careful consideration and depends on the under-floor heating zoning, and a buffer tank may be needed and detailed calculations are needed to determine the requirements to prevent heat pump cycling.
Table 1: Practical heat pump extraction rates per m2 ground
Table 2: Practical heat pump extraction rates per metre run of vertical bore hole (see EN 15450:2007 for full detail)
Figure 6 : Cold-water pre-heat applications
© Tim Dwyer and Yan Evans 2010